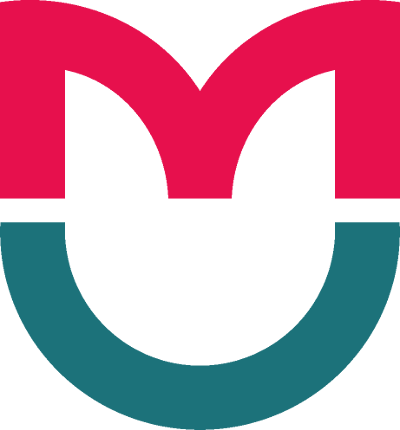
ORIGINAL RESEARCH
Effects of various mRNA-LNP vaccine doses on neuroinflammation in BALB/c mice
1 Research Center for Translational Medicine, Sirius University of Science and Technology, Sirius, Russia
2 Lomonosov Moscow State University, Moscow, Russia
3 Belozersky Institute of Physico-Chemical Biology, Lomonosov Moscow State University, Moscow, Russia
4 St. Petersburg Pavlov State Medical University, St. Petersburg, Russia
5 Institute of Cytology and Genetics, Novosibirsk, Russia
Correspondence should be addressed: Vasily V. Reshetnikov
Olimpiyskiy prospekt, 1, Sochi, 354340, Russia; ur.hepsuitnalat@vv.vokintehser
Funding: the study was supported by the Ministry of Science and Higher Education of the Russian Federation (agreement № 075-10-2021-113, project ID RF-193021X0001).
Author contribution: Kirshina AS — RNA extraction, conducting PCR; Kazakova AA, Кolosova ES, Imasheva EA, Vasileva ОО — generating genetic constructs, RNA extraction, manuscript writing; Zaborova ОV — RNA formulation in LNP, manuscript writing; Terenin IM — RNA synthesis, manuscript writing; Muslimov AR — animal experiment, manuscript editing; Reshetnikov VV — animal experiment, data analysis, preparing illustrations, manuscript wr.
Compliance with ethical standards: the study was appoved by the Ethics Committee of Pavlov First St.Petersburg State Medical University (protocol № 83 of 21 September 2022); it was conducted in accordance with the European Convention for the Protection of Vertebrate Animals used for Experimental and other Scientific Purposes (ETS No. 123, Strasbourg, 1986, with the 2006 Appendix), international convention on the humane treatment of animals (1986), Guide for the Care and Use of Laboratory Animals, 8th ed. (2010); Directive 2010/63/EU of the European Parliament and of the Council on the protection of animals used for scientific purposes (2010), Principles of Good Laboratory Practice (2016).
- Baden LR, et al. Efficacy and Safety of the mRNA-1273 SARS-CoV-2 Vaccine. N Engl J Med. 2021; 384 (5): 403–16.
- Polack FP, et al. Safety and Efficacy of the BNT162b2 mRNA Covid-19 Vaccine. N Engl J Med. 2020; 383 (27): 2603–15.
- Kon E, Elia U, Peer D. Principles for designing an optimal mRNA lipid nanoparticle vaccine. Curr Opin Biotechnol. 2022; 73: 329–36.
- Ndeupen S, et al. The mRNA-LNP platform's lipid nanoparticle component used in preclinical vaccine studies is highly inflammatory. iScience. 2021; 24 (12): 103479.
- Parhiz H. et al. Added to pre-existing inflammation, mRNA-lipid nanoparticles induce inflammation exacerbation (IE). J Control Release. 2022; 344: 50–61.
- Mu X, Hur S. Immunogenicity of In Vitro-Transcribed RNA. Acc Chem Res. 2021; 54 (21): 4012–23.
- Heil F. et al. Species-specific recognition of single-stranded RNA via toll-like receptor 7 and 8. Science. 2004; 303 (5663): 1526–9.
- Trougakos IP, et al. Adverse effects of COVID-19 mRNA vaccines: the spike hypothesis. Trends Mol Med. 2022; 28 (7): 542–54.
- Reshetnikov VV, et al. Social defeat stress in adult mice causes alterations in gene expression, alternative splicing, and the epigenetic landscape of H3K4me3 in the prefrontal cortex: An impact of early-life stress. Prog Neuropsychopharmacol Biol Psychiatry. 2021; 106: 110068.
- Bahl K, et al. Preclinical and Clinical Demonstration of Immunogenicity by mRNA Vaccines against H10N8 and H7N9 Influenza Viruses. Mol Ther. 2017; 25 (6): 1316–27.
- Liang F, et al. Efficient Targeting and Activation of Antigen- Presenting Cells In Vivo after Modified mRNA Vaccine Administration in Rhesus Macaques. Mol Ther. 2017; 25 (12): 2635–47.
- Maugeri M, et al. Linkage between endosomal escape of LNP-mRNA and loading into EVs for transport to other cells. Nat Commun. 2019; 10 (1): 4333.
- Pardi N, et al. Expression kinetics of nucleoside-modified mRNA delivered in lipid nanoparticles to mice by various routes. J Control Release. 2015; 217: 345–1.
- Hoogland IC, et al. Systemic inflammation and microglial activation: systematic review of animal experiments. J Neuroinflammation. 2015; 12: 114.
- Bilimoria PM, Stevens B. Microglia function during brain development: New insights from animal models. Brain Res. 2015; 1617: 7–17.
- Chen Z, et al. Microglial displacement of inhibitory synapses provides neuroprotection in the adult brain. Nat Commun. 2014; 5: 4486.
- Ji K, et al. Microglia actively regulate the number of functional synapses. PLoS One. 2013; 8 (2): e56293.
- Biesmans S, et al. Systemic immune activation leads to neuroinflammation and sickness behavior in mice. Mediators Inflamm. 2013; 2013: 271359.
- Buttini M, Limonta S, Boddeke HW. Peripheral administration of lipopolysaccharide induces activation of microglial cells in rat brain. Neurochem Int. 1996; 29 (1): 25–35.
- Mutovina A, et al. Unique Features of the Immune Response in BTBR Mice. Int J Mol Sci. 2022; 23 (24).
- Cunningham C, et al. The sickness behaviour and CNS inflammatory mediator profile induced by systemic challenge of mice with synthetic double-stranded RNA (poly I:C). Brain Behav Immun. 2007; 21 (4): 490–502.
- Goldstein DS, Kopin IJ. Homeostatic systems, biocybernetics, and autonomic neuroscience. Auton Neurosci. 2017; 208: 15–28.
- Burfeind KG, Michaelis KA, Marks DL. The central role of hypothalamic inflammation in the acute illness response and cachexia. Semin Cell Dev Biol. 2016; 54: 42–52.
- Rahman MH, et al. Hypothalamic inflammation and malfunctioning glia in the pathophysiology of obesity and diabetes: Translational significance. Biochem Pharmacol. 2018; 153: 123–33.
- de Git KC, Adan RA. Leptin resistance in diet-induced obesity: the role of hypothalamic inflammation. Obes Rev. 2015; 16 (3): 207–24.
- Mendes NF, et al. Hypothalamic Microglial Heterogeneity and Signature under High Fat Diet-Induced Inflammation. Int J Mol Sci. 2021; 22 (5).