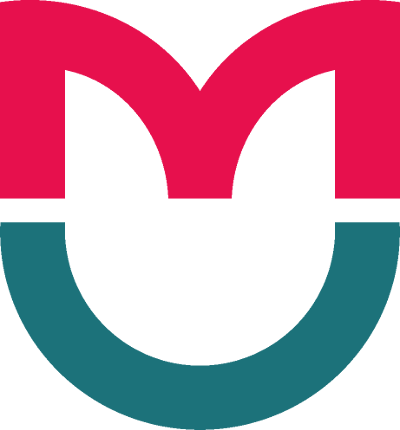
ORIGINAL RESEARCH
Activation of microglia in the brain of spontaneously hypertensive rats
1 Institute of Experimental Medicine, St Petersburg, Russia
2 St Petersburg State University, St Petersburg, Russia
Correspondence should be addressed: Valeria V. Guselnikova
Acad. Pavlov, 12, Saint-Petersburg, 197376, Russia; ur.xednay@aiirelav.avocinlesug
Funding: the study was funded by the Russian Science Foundation, project № 22-25-00105, https://rscf.ru/project/22-25-00105/.
Author contribution: Guselnikova VV — literature analysis, analysis and interpretation of the results, preparation of the manuscript; Razenkova VA — development of protocols for immunofluorescent reactions, confocal laser microscopy; Sufieva DA — histological examination of biological material, performing immunohistochemical reactions for light microscopy; Korzhevskii DE — concept development, research planning, manuscript editing.
Compliance with ethical standards: the study was approved by the Ethics Committee of the Federal State Budgetary Scientific Institution "IEM" (protocol № 1/22 dated February 18, 2022, protocol № 3/19 dated April 25, 2019), and was conducted in accordance with the provisions of the Declaration of Helsinki (2013)
Today, arterial hypertension (AH) remains one of the most significant health and social problems worldwide. According to a joint study by WHO and Imperial College London, the number of people with AH has almost doubled in 30 years (from 1990 to 2019), from 650 million to 1.28 billion [1]. The prevalence of AH in Russia, according to the ESSE-RF study (Epidemiology of cardiovascular diseases and their risk factors in the regions of the Russian Federation), was 44% in 2014 in a representative sample of patients aged 25–65 years. An epidemiological survey conducted in 2019 involving 20,607 residents of the Russian Federation, reported AH in 14,853 (72.1%) of those surveyed [2]. It is clear that AH is a widespread disease in our country, posing a serious problem for domestic health care. Despite more than a century of research, AH remains poorly treated, probably due to the complexity of the mechanisms of pathogenesis, involving many interacting factors, and our limited understanding of these mechanisms at the cellular and molecular levels [3]. Further research in this area thus looks relevant.
Being widespread in the Russian Federation, featuring inadequate treatment and control, AH remains a poorly managed risk factor for myocardial infarction, chronic heart failure, sudden death, renal failure, and ischaemic and hemorrhagic stroke. The brain is one of the target organs most early affected by AH: small-diameter vessels are the first to be affected, resulting in microbleeds, subcortical lacunar infarcts and diffuse white matter lesions, accompanied by cognitive decline [4]. It has been noted that AH is accompanied by the development of chronic neuroinflammation in the brain [5]. However, the cellular mechanisms of neuroinflammation associated with AH are unknown, and it is unclear whether neuroinflammation contributes to the progression of AH. Since inflammation in the brain is mainly caused and maintained by microglia, a detailed analysis of this cell population in the setting of AH seems relevant. New data on the functional status of brain microglia in the setting of AH may contribute to a better understanding of the molecular and cellular mechanisms underlying the development of the disease. The aim was to investigate the morphofunctional state of microglia in different brain regions of the rat in the setting of AH development.
METHODS
The SHR (Spontaneously Hypertensive Rats) strain was used as an AH experimental model. Animals of this strain, bred by K. Okamoto and K. Aoki (1963) from normotensive Wistar-Kyoto (WKY) rats, develop chronic hypertension spontaneously (without any preceding primary disease), which is a consequence of dysfunction of 1–6 genes involved in blood pressure regulation. Persistent increase in blood pressure to 190–200 mmHg in SHR rats starts from week 12 onwards [6]. The SHR line is considered the most adequate model of human essential hypertension and is widely used to study the pathogenesis of the disease and to develop new approaches to its therapy [7, 8].
Brain samples from spontaneously hypertensive male SHR rats aged 3–8 months (n = 4) were used as study material. The brain of male WKY rats of the same age was used as a control group (n = 3). Systolic blood pressure in animals was determined in the caudal artery using Sistola, a system for noninvasive blood pressure measurement in rodents (Neurobotics; Russia). Blood pressure readings were 220 mmHg in SHR rats and 85–100 mmHg in WKY rats. Brain samples were fixed in zinc-ethanol-formaldehyde using the previously described method [9], then dehydrated and embedded in Type 6 paraffin (ThermoScientific; USA). Then, 5 µm thick sections were made from paraffin blocks using a Microm HM 325 rotary microtome (ThermoScientific; USA). The obtained preparations after deparaffinization and rehydration were subjected to a heat demasking procedure by soaking in 10% aqueous sodium thiosulphate solution for 23 min.
Rabbit polyclonal antibodies to Iba1 (Biocare Medical; USA) at a dilution of 1 : 500 were used for immunohistochemical detection of Iba1. HRP Conjugate reagent from the Reveal Polyvalent HRP DAB Detection System kit (Spring Bioscience; USA) was used as a secondary reagent for the detection of primary antibodies. Peroxidase label was detected using diaminobenzidine chromogen from the DAB+ kit (Agilent; USA). After the immunohistochemical reaction, some of the slices were counterstained with alum hematoxylin.
For simultaneous detection of Iba1 and CD68, a mixture of rabbit polyclonal antibodies to Iba1 (Biocare medical; USA) at a dilution of 1 : 500 and mouse monoclonal antibodies to CD68 (Agilent; USA) at a dilution of 1 : 1000 were applied to slices. The primary antibody mixture was prepared in a 1 : 1 ratio. The secondary reagents were the mixture (1 : 1) of antibodies against biotin-labelled rabbit immunoglobulins (from R&D Systems kit; USA) and EnVision+/HRP-AntiMouse reagent (Agilent; USA) with normal rat serum added to prevent non-specific antibody binding. After incubation in the secondary antibody mixture, the slices were treated with streptavidin conjugate solution with fluorochrome Cy2 (Jackson ImmunoResearch; USA) and then with antibody solution against HRP conjugated with fluorochrome Cy3 (Jackson Immuno Research; USA).
For the negative antibody control, immunohistochemical processing of the preparations was performed without using primary antibodies, instead of which PBS was applied to the slices. There was no positive reaction in this case.
The preparations were examined using a Leica DM750 light microscope (Leica; Germany) and a LSM800 confocal laser microscope (Zeiss; Germany). A laser with a wavelength of 488 nm was used to excite Cy2 fluorescence, and a laser with a wavelength of 561 nm was used for Cy3 excitation. The images were studied using LSM Image Browser and ZEN2012 software (Zeiss, Germany).
In the quantitative testing, we evaluated the number of Iba1+ microgliocyte bodies and the area occupied by Iba1-immunopositive microgliocyte bodies and processes per 1 mm2 of nerve tissue. Measurements were made in three 270 × 203 µm fields of view for each case in each study area (cortex, striatum, white matter, subfornical organ) using Fiji morphometric analysis software (ImageJ). The number of cell bodies was counted using the Cell Counter plug-in. The area occupied by microgliocytes was estimated after channel separation (DAB/hematoxylin) using the "Color Deconvolution" option followed by application of the "Threshold" and "Measure Particles" options [10]. Statistical analysis was performed with GraphPad Prism 8 software (GraphPad Software; USA). Data were presented as mean ± SEM. The data were compared using the Mann–Whitney test. The criterion to deem the differences significant was p < 0.05.
RESULTS
After performing an immunohistochemical reaction for the calcium-binding protein Iba1, immunopositive cells were detected in all brain regions studied. The reaction product was concentrated in branching cells, which have a typical microglia structure. A morphological diversity of Iba1+ cells was observed depending on their localization within the brain. In the cortex of the control group animals (WKY), typical ramified microglia without signs of activation were present (fig. 1A). Iba+ cells in this case were characterized by a small soma and the presence of long thin processes branching in different directions. The cell body was often not in the slice plane, resulting in the visualization of only parts of microglial cell processes in the form of immunopositive strands and/or dots in some cortical areas. The immunopositive elements detected were evenly distributed within the cortex (fig. 1А). In similar cortical areas of spontaneously hypertensive rats (SHR), microglia had a number of differences (fig. 1B). As in the control group, the immunopositive cells in this case were evenly distributed without forming clusters, but the density of Iba1+ cells was visually significantly higher. We observed an increase in the size of the microglial cell body and thickening of their processes, which demonstrated a high branching rate (fig. 1B). Such morphological features were characteristic of microglia in all layers of the cerebral cortex of spontaneously hypertensive rats.
The average area occupied by Iba1+ microglia in the cerebral cortex of the control group animals was 27,547 ± 3,100 µm2/mm2, and that with spontaneously hypertensive rats — 18,790 ± 2,717 µm2/mm2 (p = 0.2). The number of Iba1+-microglial cell bodies in WKY rats was 89.56 ± 10.86 cell bodies/mm2, and in SHR rats — 130.1 ± 19.9 cell bodies/mm2 (p = 0.2).
In the striatum of control animals, as in the cortex, a typical ramified microglia were found (fig. 1B). At the same time, the thickness of microglial processes was greater compared to the cortex of control animals, making the complex branching of microglial outgrowths in this localization more visible. As in the large hemisphere cortex, microglia were evenly distributed in the striatum, without forming clusters. Cell bodies were small, rounded or oval in shape and not always in the slice plane. Microglia were localized predominantly in the grey matter of the striatum, and only short sections of these cell processes were visible in the white matter (fig. 1C). The average area of Iba1+ microglia was 33,887 ± 3,944 µm2/mm2, the number of Iba1+ cell bodies was 111.7 ± 14.31 cell bodies/mm2. In the striatum of spontaneously hypertensive rats, the shape and distribution of microglia did not differ from that of control animals, but there was an increase in the size of these cell bodies and a thickening of their processes, which demonstrated a high branching rate (fig. 1 D). The average area of Iba1+ microglia in this case was 21,516 ± 2,589 µm2/mm2, and the number of cell bodies was 146.3 ± 12.68 cell bodies/mm2, which had no statistically significant difference to the control group (p = 0.2).
In the subcortical white matter of control animals, spindle-shaped Iba1+ cells were present, with their bodies and processes oriented along the nerve fibers (fig. 1D). The microgliocytes detected were characterized by the presence of one or two long unbranched or weakly branched offshoots arising from different poles of the cell body in opposite directions (fig. 1D). In spontaneously hypertensive rats, an increase in body size and thickness of Iba1+ cell processes was observed in the subcortical white matter region. We found not only spindle-shaped microglia but also cells with three processes extending from the body and penetrating white matter fibers in different directions. No increase in the intensity of branching was observed (fig. 1E). The mean area of Iba1+ microglia in the white matter in control animals was 11,791 ± 4,540 µm2/mm2; in spontaneously hypertensive rats it was 9,208 ± 1,368 µm2/mm2 (p > 0.9999). The number of Iba1+ microglial cell bodies was 8107.7 ± 26.97 cells/mm2 in WKY rats and 111.7 ± 2 cells/mm2 in SHR rats (p = 0.6).
A large number of Iba1-immunopositive cells, distributed relatively evenly throughout the organ, were present in the subforonic organ of the control group (fig. 1G). The morphology of microglia in this region of the brain differed significantly from the typical ramified morphology characteristic of normal microglia. Most of the Iba1+ cells present in this locus were uni- or bipolar spindle-shaped. Their processes demonstrated very little branching (often no branching) and lacked a unified direction. Within the subfornical organ, there were also single unprotected microgliocytes characterized by hypertrophy of the bodies and the absence of distinct processes (fig. 1G). In the subfornical organ of spontaneously hypertensive rats, all detected Iba1+ cells had not many processes. They were characterized by marked hypertrophy of the bodies and the presence of few short, thick, unbranched ramifications (fig. 1H). The mean area of Iba1+ microglia in the subfornical organ in control animals was 37,831 ± 8283 µm2/mm2, and in spontaneously hypertensive rats it was 45,783 ± 4,318 µm2/mm2 (p = 0.4). The number of Iba1+ microglial cell bodies in WKY rats was 337.4 ± 8.84 cells/mm2, in SHR rats 465.4 ± 27.05 cells/mm2 (p = 0.1).
After performing an Iba1/CD68 double immunofluorescence reaction, it was observed that in all brain regions examined, the majority of cells visualized contained Iba1 protein, evenly distributed in the bodies and processes (fig. 2; green fluorescence). The morphology of these cells in each examined area corresponded to that described in the immunohistochemical reaction to the Iba1 protein for light microscopy. When the preparations were analyzed under low magnification, the CD68 protein appeared to be completely absent from these cells. However, when analyzed under high magnification, CD68 was detected in the cytoplasm of some cells as local clusters (fig. 2B; the yellow color is the result of colocalization of green and red signals). The size, the number and the distribution of CD68 clusters in the cytoplasm varied greatly between individual microglial cells, but no dependence of these parameters on cell localization was found. Also, there was no increase in the content and/or change in the distribution of CD68 protein in spontaneously hypertensive rats compared to control group rats in any of the brain regions studied.
In addition to microgliocytes containing large amounts of Iba1 and local accumulations of CD68 in the cytoplasm, all analyzed brain regions showed the presence of single cells with high CD68 content (fig. 2B; arrowhead). The CD68 protein was detected in the cytoplasm of these cells as fine granular structures that gave the cytoplasm a granular appearance (fig. 2B; red fluorescence). The Iba1 protein within the cytoplasm of such cells was present in small amounts (as dot inclusions) or undetectable amounts. Cells high in CD68 were present in the brain of both normotensive and spontaneously hypertensive animals, and were found in all areas studied. They differed a lot from typical microglia in morphological aspect, being oval or elongated in shape, with no branching processes and occurred in most cases perivascularly, rarely within the parenchyma.
DISCUSSION
The study now discussed provides morphological evidence for chronic microglia activation in different rat brain regions in the setting of AH development. Statistical analysis revealed a tendency for the number of microglia in the brain to increase in SHR rats compared to the control group. However, it has not been possible to demonstrate statistically significant differences in the analyzed indicators given the material available, which may be due to insufficient sample size. There is also a marked scattering of indicators among the animals in the control group. This may be due to the fact that WKY rat strain, widely used as a control for the SHR line, has a number of features related to the neural tissue condition. For example, in a recent study we observed that, unlike in Wistar rats, signs of Kolmer Iba1+ cell activation can be observed in the brain of WKY rats, and supraependymal CD68+ and Iba1+ rounded cells are present in the cerebral ventricular cavity. This is indicative of a hematoliquor barrier damage and activation of monocyte-macrophage cells in WKY rats [11]. Together with our findings in this paper, this raises questions about the adequacy of using WKY rats as a control group in neurobiological studies.
It has previously been reported that SHR rats show a moderate state of microglia activation in the medulla oblongata [5]. Expression of the metabotropic purinergic receptor P2Y12 and fractalkine receptor CX3CR1 genes was observed to be reduced in SHR rats compared to normotensive WKY rats, which correlated with the phenotypic signs of activation, such as lower microglia cell density in the medulla oblongata and shorter microglial processes [5]. In addition, hypertrophy of microglia was shown in the deep cortical layers of 35-week-old SHR rats, indicating its activation [12]. Microglia activation in this study was further confirmed using flow cytometry, which showed a significant increase in CD11b expression in the brain microglia of SHR rats. In addition to this evidence of microglia activation in several brain regions in spontaneously hypertensive rats, we obtained evidence of microglia activation in the cortex, striatum, white matter and subfornical organ of SHR rats compared to WKY rats of the same age. Interestingly, the most pronounced morphological signs of activation were exhibited by microgliocytes within the subfornical organ. We noted earlier that normally microglia in this area is in a pre-activated state, which may be due to the absence of a blood–brain barrier here [13]. From the perspective of these data, it seems logical that under the influence of additional stimulation associated with arterial hypertension, microglia of the subfornical organ more readily switch to an activated state compared to microglia in other brain regions, where microglia are normally represented by an inactive (ramified) form.
The role of microglia activation in AH, depending on localization, is still an open question. It has been shown that targeted 'silencing' of microglia effectively reduces blood pressure and neuroinflammation in mice with experimental hypertension. Conversely, adoptive transfer of activated microglia would predispose recipients to hypertensive stimulants. Taken together, these results indicate that microglia are a key cellular element in the neurogenic regulation of hypertension [14]. Further research into the contribution of microglia and neuroinflammation (mediated by the latter) to the pathogenesis of AH may help identify new molecular targets for targeted therapies for this disease.
The fact that microglia are activated in the setting of AH may have important therapeutic implications. For example, chronic AH has been found to be a risk factor for Alzheimer's disease, the most common neurodegenerative disease, which currently cannot be prevented or treated effectively. Neuroinflammation caused by glial cells is thought to be one possible mechanism linking hypertension and an increased risk of developing Alzheimer's disease [15]. Moreover, the available experimental evidence suggests that glia activation and neuroinflammation can be reversed by the use of different classes of antihypertensive drugs. These studies suggest that antihypertensives may be effective in Alzheimer's disease not only because of their ability to affect blood pressure, but also because of their anti-inflammatory effects [15].
Microglia activation is known to be accompanied by an increase in phagocytic activity of these cells. Phagocytosis, carried out by microglia, is critical for the normal functioning of the central nervous system at all stages of its development. During embryonic and early postnatal development, microglia clean the developing brain of apoptotic neurons and oligodendrocytes through phagocytosis. In the adult brain, microglia will normally phagocytize fragments of dying cells as well as inactive synapses, contributing to the regulation of synaptic plasticity and the maintenance of neural tissue homeostasis. During ageing, microglia will phagocytize the remains of dying neurons and collapsing synapses. The phagocytic activity of microglia is increased when pathology develops: in diseases such as Alzheimer's or multiple sclerosis, microglia can phagocytose not only dying neurons, but also β-amyloid and myelin [16, 17].
One of the marker proteins for judging the phagocytic activity of cells is the transmembrane receptor protein CD68. It is localized in the lysosomal and endosomal membranes of monocyticmacrophage cells and microglia. CD68 increases dramatically in inflammatory conditions, so CD68 has traditionally been considered a marker of activated microglia [18]. Based on these findings as well as morphological evidence of microglia activation in different brain regions in spontaneously hypertensive SHR rats, we expected to see an increased content of CD68 protein in microglia in SHR rats compared to control group. Unexpectedly, we found no such increase in any of the brain regions studied. This suggests that activation of microglia against the background of AH is probably not associated with an increase in phagocytic activity of these cells, and may have a regulatory (secretory) nature.
An interesting finding in our research was the discovery of a population of CD68-immunopositive cells with very low levels of Iba1 protein. The detection of Iba1–/CD68+ cells in the human brain has previously been reported by other authors [19]. They noted that lesions in the deep subcortical structures of the brain result in an increase in microglia containing CD68, a transformation of branched microglia into amoeboid microglia and a loss of Iba1 expression. According to our quantitative assessment, Iba1–/CD68+ cells account for about 32.5% of total microglia in deep subcortical brain lesions [19]. It has also been noted that in Alzheimer's disease, the development of dementia is positively correlated with CD68 levels and negatively correlated with Iba1 levels, and that different microglia populations expressing these two proteins can coexist in the brain [20]. Whether the Iba1–/CD68+ cells detected by us and other authors are a new type of microglial cells or a special population of macrophages needs further investigation.
CONCLUSIONS
The development of chronic AH in SHR rats involves activation of microglia in the cerebral cortex, striatum, subcortical white matter and subfornical organ. The subfornical organ microglia in normotensive rats is in a pre-activated state. Activation of microglia in the organ increases with the development of AH. Activation of microglia in AH is not accompanied by an increase in phagocytic activity of these cells. Microglia is involved in the development of neuroinflammation in AH and may therefore be a potential target for targeted pharmacotherapy for this disease.