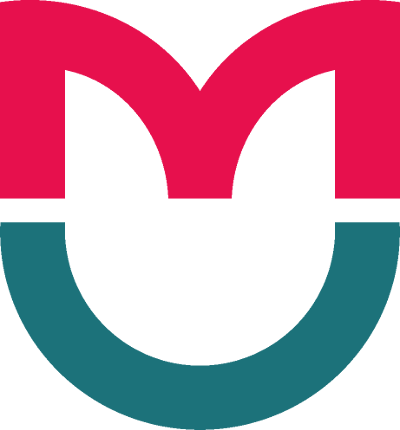
This article is an open access article distributed under the terms and conditions of the Creative Commons Attribution license (CC BY).
ORIGINAL RESEARCH
Possible links of wildfires with oncological diseases of children and adults in the Russian Far East
1 Khabarovsk Federal Research Center, Far Eastern branch of the Russian Academy of Sciences, Khabarovsk, Russia
2 Tsinghua University, Beijing, China
3 The Southern Scientific Centre of the Russian Academy of Sciences, Rostov-on-Don, Russia
4 The University of Hong Kong, Hong Kong
5 National Medical Research Radiological Centre of the Ministry of Health of the Russian Federation. Moscow, Russia
6 Peoples’ Friendship University of Russia, Moscow, Russia
7 Burnazyan Federal Medical Biophysical Center of the Federal Medical and Biological Agency, Moscow, Russia
8 Far Eastern State Medical University, Khabarovsk, Russia
Correspondence should be addressed: Sergey K. Pinaev
Muravyova-Amuskogo, 35, Khabarovsk, 680000, Russia; ur.liam@veanip
Acknowledgments: we thank Professor N.E. Kosykh from Far Eastern Medical University for their help in collecting data on the updated incidence of malignant neoplasms in children in Khabarovskij Kraj. We acknowledged Olga P. Gretsova for preparing the data for analysis. S. Venevsky acknowledges the support from the Russian State Assignment of the Federal Research Centre of the Southern Scientific Centre of the Russian Academy of Sciences (SSC RAS) (122013100131-9) and Department of Earth System Sciences of Tsinghua University.
Author contribution: Pinaev SK — author of the general idea and the main design of the study, collection and analysis of data, and formation of hypotheses; Venevsky S — developed method of spatial and non-linear temporal analysis, presentation and interpretation of results of this analysis and made conclusions; Chakov VV — collection and analysis of information about the fire situation in the Far Eastern Federal district on the basis of data presented in the public domain; Linwei Tian — run ARIMA analysis; Chizhov AYa, Peng Gong, Pinaeva OG — participated in the planning of the study and discussion of the results; Kaprin AD, Venevsky S — Participation in research planning and formation of data on CI based on the national cancer registry of Russia; Venevsky S, Pinaev SK — wrote the manuscript.
Growth of cancer incidence (CI) worldwide is related not only to the growth and aging of the population [1], but as well to the growth of some environmental risks associated with socioeconomic development and climate change. Studies at large spatial scales — the global, national, and regional levels — are necessary to understand the relationship between CI and environmental conditions. Such studies are conducted [2], but their number is still not sufficient.
Russia had a slightly smaller increase in age-standardized CI rates in both sexes for all cancers in 195 countries or territories from 2005 to 2015 (0% to 10%) in comparison with other countries with high Human Development Index (HDI) (10% to 20%) [3]. Inter-regional comparison of CI rates in Russia demonstrates, however, that not only is the aging of the population in Russia is responsible for cancers, but also other factors which likely include environmental air pollution. Indeed, a comparison of all cancers age-standardized CI in the Russian Federation in total with the one in the Far East Federal District (FEFD) in 2019 revealed that level of all cancer incidences in FEFD is more than in Russia (269.15 against 249.54 per 100,000) [4]. Meanwhile, average age of population is lowest in FEFD in relation to the other federal districts (and 2 years lower than for the entire Russia [5]). Wildfires could be one of the factors promoting cancers in FEFD. Indeed, remote sensing analysis confirms that areas burnt in forested areas in Russia had a significant increasing trend from 2000 to 2016 [5] which continues until now, presumably due to ongoing climate change. The major input to the burned areas comes from the Far East and East Siberian Federal Districts; major ignitions occur near large cities in these districts, especially near the southern border of the Asian part of the Russian Federation [6]. Wildfire smoke contains particulate matter PM2.5/ PM4 and carcinogens, such as benzene and formaldehyde, which have been shown to increase cancer risk in American firefighters [7]. Air pollution in FEFD [8] and tobacco consumption are close an average for Russia here [9]. Thus, exposure to wildfires smoke of population in southern settlements of FEFD may explain excess of CI in the district.
Smoke from wildfires was found to have variety of negative effects to human health [7], increasing both mortality and morbidity to cardiovascular, respiratory and other diseases. Particulate matter in smoke were found to be most important stressors, affecting human health through pulmonary oxidative stress, which can cause cell death or DNA damage, and inflammation [10]. Both oxidative stress and inflammation are major modulators of cancers, thus, airborne particulate matter was classified by the International Agency for Research on Cancer as carcinogen of Group I for lung cancer and potentially to other cancers [11]. Thus, long term exposure of humans to PM and other carcinogens in wildfire smoke may affect cancer mortality and/or cancer incidence.
For the first time V.A. Dobrykh and T.A. Zakharycheva pointed out the connection between forest fires and malignant neoplasms of the respiratory organs [12]. Subsequently, this was confirmed by researchers from Canada, who also established the effect of natural fire smoke on the incidence of brain tumors [13]. We have previously reported on the relationship between forest fires and the frequency of various neoplasms in children and adults [14, 15].
Cancer is a complex phenomenon. It represents a class of diseases that manifests in different forms and presentations. Cancer destroys different target tissues and has a wide variety of etiologies. In addition, age-specific global contributions of cancer types to the total CI are quite different for young children up to 4 years, children/teenagers up to 14 years and the entire population [3]. Thus, analysis of at least two age population groups (children/teens up to 14 years and the entire population) for CI in conditions of airborne pollution can provide a full picture of relationships between different cancer types and wildfires. Here, we studied spatial and temporal associations of wildfires with cancer incidence for the period 1992–2019 years in two age population groups (children/teenagers up to 14 years and the entire population) for the Far East Federal district in Russia prone to wildfires using an annual total number of fires as a proxy for long term exposure of humans to smoke. We also analyzed the relationship between the number of fires (NF) and CI in one age cohort of young children (0–4 years) for the period 1972–1986 in Khabarovskij Kraj (central AU of the FFED until 2018). This study has an objective a) to investigate if statistically significant temporal associations exist between the incidence of different types of cancer and NF in the Far East Federal district in one age cohort (young children) and in two population groups (children/teens 0–14 year and the entire population) for NF and CI and b) to estimate what are time lags in these associations and c) to find out what age group is most vulnerable to impact of wildfires. Existence of large geographical regions, for which statistical relations between wildfires and oncological diseases can be found, was a major hypothesis of this study.
METHODS
Study area
The Far Eastern Federal District (FEFD) is sparsely populated district (area 6.9 million km2) with a variety of climatic, vegetation, and topographic features. The majority of the FEFD population lives in large cities in the south of the district. Seven administrative units (AUs) of the FFED with developed medical registration system were taken for this study (fig. 1).
Definition of spatial regions of analysis and cancer classification used in this study
Spatial regions of analysis, where possibility of statistical relationship between wildfires and oncological diseases was investigated, were designed based on the set of seven AUs of the FFED. Each spatial region of analysis was a combination from one, two, etc., up to the seven AUs. Existence of statistical relationship “wildfires/oncological diseases” was checked for the all 127 spatial regions (C17+ C27 + C37 + C47 + C57 + C67 + C77 = 127), where Cm7 is a number of possible combinations of m from the seven AUs) for two age groups “children/teenagers up to 14 years” and “the entire population”. Difference in etiology of cancers was not considered for comparison of the two age groups. Analysis of all cancer incidences in this study for young children up to 4 years, children/teenagers up to 14 years and the entire population is done for major cancer types for children for the unity of approach The major cancer types for children in this study mainly followed the international classification of malignant tumors [16]. Five major malignant tumor types (leukemia (LK), Hodgkin lymphomas (HL), nonHodgkin lymphomas (NHL), central nervous system tumors (CNS), and soft tissue sarcomas (STS)), which constitute major child cancer cases both in highly industrialized countries, like Russia, and in the world (approximately 75%) [16].
Possibility of existence of statistical relationship “wildfires/ oncological diseases” was studied in more details for the age cohort of young children (0–4 years) in Khabarovskij Kraj (central AU of the FFED until 2018). The choice of age cohort of young children (0–4 years) for an additional analysis was done because tumors of young children usually are started to develop already in prenatal period [17], while impact of carcinogens at the level of entire population may take several years or even decades [18]. Three additional embryonal types of cancer (retinoblastoma, neuroblastoma, nefroblastoma) and five major benign tumors (BT) in young children, namely hemangiomas, lymphangiomas, teratomas, soft tissue tumors, and papillomas, were included additionally to the five common cancer types for young children (0–4 years)
Number of fires as a proxy for human exposure to wildfire smoke in FEFD
It was possible to use either annual burnt area in AUs data or annual number of fires in AUs data, because the fine resolution data on spatial and seasonal distribution of fire hotspots were limited by the year 1996 [19] and spatial-temporal CI data are available only at annual time step. Canadian researchers [20] suggested to use annual burnt areas at a distance 30–50 km from settlements for an analysis of relationships “wildfires/ oncological diseases. However, it is not possible to use total areas burnt in AU (as a proxy of population exposure to smoke from wildfires in FEFD due to uneven location of settlements in FEFD (all major settlements are situated here to the south of 55 NL). Oppositely, total number of fires in administrative unit in FEFD is a good proxy for long term exposure to carcinogens (mostly PM2.5). Indeed, they highly correlate with areas burnt for five southern populated AUs of FEFD (see Supplementary Analysis of number of total, human and lightning fires in administrative units of Russian Far East against areas burnt in 1990–2014) and number of human ignited fires (situated almost exclusively near the cities) is almost equal to number of total fires in these five AUs. Administrative units with large remote areas (Republic Sakha and Magadan Oblast) have total area burnt mainly correlated with number of lightning fires which are few, while number of human fires (located in proximity of cities) is still correlated to total number of fires in these two remaining AUs. The fires in southern populated areas are mainly understory small fires (92% on average for entire FFED, see Supplementary Analysis of number of total, human and lightning fires in administrative units of Russian Far East against areas burnt in 1990–2014), which are producing major emissions (including particulate) reaching population, while large and long wildfires are going mainly in hardly accessible areas in the North. Thus, this study adopts total number of fires in AUs as a proxy for population long-term exposure to wildfire carcinogens emissions. Annual total number of fires were normalized to an average value for the entire period 28-years period in each of the seven AUs for inter-comparison and weighted to the population numbers in AUs when administrative units were combined together.
Data
Data on CI (persons with cancer for 100 000 persons) for five major cancer types in seven listed oblasts (or AUs) in two age groups (children and teenagers 0–14 years and the entire population) for the 28-year period (1992–2019) were used in this study (see Table 1 in Supplementary Data)). Data consist of the annual number of all cancer cases by type registered for one of the population groups in an AU to hundred thousand persons in this group (e.g., for age population group: children/ teens 0–14 year in the year 1992, this is a number of cancer cases by a type for persons of the 1978–1992 birth years in the AU divided by the total number of children/teen 0–14 years old in the year 1992, expressed in 100 000 persons, in this AU). The data were extracted from federal statistical data using the Informational Analytical Data Base Management System (Russian state software registration number 2011617155) in the Russian Center for Information Technology and Epidemiology Studies in Oncology named after Pierre Herzen (author Olga P. Gretsova, 2020). Annual cancer incidence values were normalized to a maximum value for the entire period 28-years period for the seven AUs for each of the five cancer types for inter-comparison purposes.
We also analyzed data on tumor incidence in young children 0-4 year. Our data were collected for the largest city of FEFD, Khabarovsk (over 613 thousand inhabitants), the capital of FEFD until 2018, with the most developed medical registration system, and for Khabarovskij Kraj (central AU until 2018). The data were presented for the total number of births for years 1976–1986 in Khabarovsk for benign tumors (see Table 2, Supplementary Data) and for years 1972–1988 for Khabarovskij Kraj for malignant tumors (see Table 3, Supplementary Data).
Number of fires by the seven AUs (Table 4, Supplementary Data) was downloaded from the Russian Ministry of Forestry dataset.
Statistical analysis
Annual values of CI by the five cancer types and NF in the AUs were normalized for their maximum values over the 28-year period for all of the seven AUs. Such a normalization allows comparison of amplitudes, trends, number of cycles and autoregressive features of time series. This approach does not allow to estimate factor dependence of CI by NF (this was not our objective), but allows to minimize influence of different regional co-factors of oncological diseases by statistical analysis.
Firstly, we checked if statistically significant difference of medians for normalized NF and CI by the five cancer types within each of the two population age groups for the seven AUs was observed. This was done using Krsukal–Wallis test with a threshold α = 0.05. Similar analysis was conducted after the time series of CI and NF were weighted to a ratio of total number of population in an AU to the total number of population in all seven AUs. Additionally, we checked if statistically significant difference between CIs for the five cancer types (also for the weighted by total number of population time series) was observed within each from the seven AUs using Krsukal–Wallis test with an α = 0.05.
The temporal dependence of CI (normalized) on NF (normalized) for a long time series (28 years) was studied using ARIMA models [20], which were applied as for a description of wildfires dynamic characteristics [21], so for description of CIs [22]. An ARIMA model was first applied to the NF (assumed to be a predictor) with a time lag of 0–6 years (upper border of the time lag is approximately twice as the time for complete renewal of organism’s cells). Afterwards, the ARIMA model was fit to the CI (by type). The resulting fitted series (predicted against dependent variables) were cross-correlated to identify associations. A statistically significant association between NF with a lag from 0 to 6 years and a CI by type was prescribed to have a p-value less than 0.05 and marginally statistically significant association was prescribed to have a p-value between 0.05 and 0.1. The Ljung-Box Q test was applied to statistically significant associations to ensure that the residuals series were white noise, which indicates the goodness of the resulting association. Statistical associations “NF/CI” were studied within two age groups (children/teens 0–14 years and entire population) for the 127 sets of AUs combinations (C17 + C27 + + C37 + C47 + C57 + C67 + C77 = 127 see fig. 2). Cancer incidence time series for each cancer types and NF time series for all AUs within one combination were pooled together making five CIs time series and one NF time series for 28 years in this combination. Pooling in a single time series for CIs from the initial time series was performed in two ways: 1) annual mean CI for several pooled AUs was calculated for each year and 2) annual CIs were weighted by ratios of population in each from AUs to total population within the combination before summation for each year. The two methods of calculation for pooling together of CI time series produce similar results, but some difference in value exists (not shown). Pooling in a single time series for normalized NF from the initial time series was done with weighting of annual values by ratios of population in each from AUs to total population within the combination before summation for each year. One hundred twenty-seven combinations were divided into seven classes (with one to seven combined AUs in a class) and list of correlated associations “CI/NF” in sense of ARIMA modelling with their regression coefficients, p-values and time lags was identified within each class (fig. 2). Bonferroni-Holm approach was applied to estimate a probability of type I error when conducting multiple ARIMA calculation within each of the two age groups for the five types of cancer.
A short time series of CI by type were studied using linear regression and correlation analyses for an age cohort “children 0–4 years”. We considered the possibility of time lags in pollution stress for tumor development and conducted linear regression and correlation analysis of CI against NF with a time lag relatively to the birth year of the children (–3, –2, –1, 0, 1, 2, 3 — in years). Such a linear statistical analysis was applied to analyze the incidence of benign tumors in young children cohorts 0–4 years old born between 1976–1986 against NF in Khabarovsk. A similar analysis was performed for malignant tumors in cohorts born between 1972–1988 for the entire Khabarovskij Kraj.
We generalized our analysis of temporal relationships between CI and NF by making of histograms for number of “CI/NF” statistical associations by the five cancer types within each of the two age groups in the FFED with confidence tresholds α = 0.1 and α = 0.05. Values of number of “CI/NF” statistical associations were normalized to the maximum value for the five cancer types to get a relative strength (RS) of relationship between NF and CI by the five cancer types within each of the two age groups for the two values of the threshold α.Hystogram for the coefficient of determination of linear regression CI against NF for the all types of cancer and benign tumors for children 0–4 years for the central AU of the FFED was used for generlaziation of the linear statistical analysis.
RESULTS
Comparison of distributions of normalized values of cancer incidence and number of fires for administrative units of Far Eastern federal district
We found that the median values for normalized CI for the five cancer types in each of AU of FEFD had statistically significant differences (p ˂ 0.05) as for the age group “children and teenagers 0–14 years”, so for “the entire population” group (fig. 3A–D) both in the case of normalized units and in the case of normalized units, weighted by number of population in an AU to the total population in the AUs. Medians of almost all CIs and NF (elven for the normalized units and twelve (all) for the normalized weighted units) had statistically significant differences for the group of entire seven AUs within the two age groups. An exception was a case of leukemia in the age group “children and teenagers 0–14 years” for the normalized units (p = 0.0536 close to the threshold). Weighting by number of population in an AU to the total population in the AUs made better subdivision of both CIs and NF by the AUs and larger statistical significance of differences as for the age group “children and teenagers 0–14 years” (fig. 3A, C), so for “the entire population” group (fig. 3B, D). Intervals between low and upper quartiles of CI distributions were (as a rule) larger for the age group “children and teenagers 0–14 years” than for age group “the entire population”. Distributions of CIs differed from NF distributions most profoundly as by medians, so by lower and upper quartiles, for Kamchatskij Kraj and Sakhalin Oblast’.
Time series analysis of relationship between number of fires and cancer incidence by cancer types and spatial regions with different administrative units combinations
Statistically significant associations with marginal threshold α = 0.1 between normalized NF and normalized CI time series by different cancer types and by two pooling methods with a lag from 0 to 3 years, when controlled for probability of type I error during multiple ARIMA calculations, were found for 27 pooled together combination sets of AUs within the two age groups “children/teens 0–14 years” and “entire population”. Statistically significant relationships (α = 0.05) were found for eight spatial areas ((see Supplementary List of spatial clusters of ARIMA associations between number of fires and cancer incidence by types with regression coefficients and p-values with statistical significance α = 0.1 and α = 0.05 after Holms– Bonferroni correction) ). Majority (six from eight) statistical relationships NF/CI with α = 0.05 where found for pooling together AUs method with weighting to number of population in AUs. Kamchatskij Kraj and Sakhalin Oblast, where distributions of NF and CIs are considerably different (see above) were not met in any from 27 spatial regions with NF/CI relationships. The four most populous AUs pooled together (Primorskij Kraj — Khabarovskij Kraj — Amurskaja Oblast — Respublika Sakha) showed the largest number of ARIMA-based statistical associations between NF and CI (six) with α = 0.1 (two from these six had α < 0.05). However, adding to a pooled combination of an administrative unit with a smaller population may distort the previously found associations. Negative values of ARIMA-based regression coefficients of annual NF against annual CI were found in some cases (see Supplementary List of spatial clusters of ARIMA associations between number of fires and cancer incidence by types with regression coefficients and p-values with statistical significance α = 0.1 and α = 0.05 after Holms–Bonferroni correction)). These negative regressions coefficients were found for the age group “children/teens 0–14 years” for leukemia (only for time lag 2 years, not for other lags), CNS tumors (lag 3 years) and STS tumors (lag 2 years) and for the age group “entire population” for leukemia (only for lags 2 years and 3 years, not for other lags). We found with ARIMA analysis that time lags for statistical associations between NF and CI are rather independent from geographical area for a taken cancer type. Time lags lay between zero (NHL for children) and 3 years (CNS tumors for children/teens 0–14 years), but mostly they are equal to 2–3 years.
Results of linear analysis of statistical dependencies between number of fires and benign, embryonal, and malignant tumor incidence by type in the age cohort: young children up to 4 years
Positive significant correlations between NF and tumor incidence in the age group: young children 0–4 were found for the period 1972–1986 in Khabarovskij Kraj/Khabarovsk. Correlations were observed for all three groups analyzed: for benign tumors (3 from 5 analyzed 0.66 < R2 < 0.84; 0.003 < р < 0.014), embryonal tumors (2 from 3 analyzed 0.54 < R2 < 0.74; 0.037 < р < 0.046), and malignant tumors (3 from 5 analyzed 0.533 < R2 < 0.73; 0.009 < р < 0.036) (fig. 4).
Hodgkin lymphomas had largest coefficient of determination R2 for linear regression between NF and CI for young children up to 4 years. Generally, R2 is higher for benign tumors than for malignant tumors for this age cohort.
Both prenatal and postnatal influences of fires were found for the age cohort: 0–4 years, which is seen from the time lags (see Supplementary Linear Statistical Analysis), which are close to the ones from ARIMA-based analysis for certain cancer types.
Cancer types in relation to wildfires
Relative strength (RS) of relationships (from 0 to 1) between normalized NF and CI, summed over all five types of cancer, was larger for the age group “entire population” as for the threshold α = 0.1 (fig. 5A), so for the threshold α = 0.05 (fig. 5B). Leukemia and non-Hodgkin lymphomas had the largest cumulative input to RS by both thresholds.
DISCUSSION
Statistical analysis of time series of CI by the five cancer types against NF confirms existence of statistical associations CI/NF in spatial regions, consisting from combinations of the five AUs of FEFD, excluding Kamchatskij Kraj and Sakhalin Oblast. We speculate that absence of statistically significant relationships between CI and NF in spatial regions which include Kamchatskij Kraj and Sakhalin Oblast, can be explained by maritime climate in these AUs, which determines smoke pattern from wildfires here. This hypothesis, as well as a hypothesis of existence of other large scale environmental spatial determinants, setting an absence or an existence of statistically significant relationships between CI and NF, should be studied further on.
Spatial regions, consisting from the AUs with largest population numbers, demonstrate the largest number of statistically significant CI/NF relationships. This can be explained a) by bigger size of samples and/or b) by better medical information systems in AUs with larger number of population. Clarification of influence of demographic and logistic spatial reasons for existence/absence of CI/NF relationships is a topic for further studies as well.
It can be assumed that negative ARIMA regression coefficients, observed in some spatial regions of analysis for some cases of cancer, are set by absence of seasonality in CI data. It is known that seasonality of CI data is related to non-sufficient registration of oncological diseases in periods of summer vacations [23]. It may be assumed that oncological diseases develop under carcinogens from smoke of large fires in summer of previous year, but get registered in a cold time of next year (i.e. 0.5 years are added to time lag, which should be rounded by adding 1 year ). An example of such a situation presumably can be a result of ARIMA regression for leukemia in the age group “children/teens 0–14 years” in the spatial region, consisting from Primorskij Kraj, Khabarovskij Kraj and Sakha (Yakutia), (see Supplementary List of spatial clusters of ARIMA associations between number of fires and cancer incidence by types with regression coefficients and p-values with statistical significance α = 0.1 and α = 0.05 after HolmsBonferroni correction). ARIMA regression coefficient in this case is negative for the time lag 2 years, but positive for the time lag 3 years. It can be explained most likely, by late registration of part of leukemia incidence cases, developed in the summer of year 2, in the beginning of winter of the year 3. This hypothesis, however, requires further investigation.
We found the stronger association of NF with CI for the age population group “entire population” for majority from five cancers in terms of RS (fig. 5). There can be several reasons: 1) pure demographic reason, i.e. a result of longer exposure of “entire population” group to smoke carcinogens due to larger age period within the group; 2) gerontological reason, i.e. due to inclusion in this group elderly population prone to many diseases including cancers; 3) socio-economic, i.e. result of influence of cofounding factors, like smoking of cigarettes or long-term exposure to industrial and/or agricultural carcinogens of some part of “entire population”. Synergetic impact of cofounding particulate emissions to cancer (e.g. particulate emission from heating in winter in FEFD [24]) and joint impact of heat wave and smoke of wildfires (e.g. example of Moscow fires in 2010 [25]) should be considered in future,
There is wide experimental and observed evidence of the carcinogenic impact of smoke from wildfires on humans (e.g. [7]). Intrusion of carcinogenic chemicals and particles of smoke into the blood leads to oxidative stress, an integrative vector for environmental impacts on organisms, which in turn promotes the hallmarks of benign and malignant tumors, such as inflammation and genetic variation caused by mutations and direct chromosome damage [26]. Three blood cancers (leukemia, nonHodgkin lymphoma, and Hodgkin lymphoma) most likely had the strongest associations with wildfires, not only because blood is an immediate agent of smoke intrusion, but also because oxidative stress interacts with other negative external impacts on humans. For example, the dependence of non-Hodgkin lymphoma on environmental factors and certain lifestyles has been well studied [27]. It was found that environmental factors that increase viral exposure or reaction to viruses (from all HIV found mostly by the entire population) pose a risk of non-Hodgkin lymphomas. Wildfires most likely affect non-Hodgkin lymphomas by weakening an individual’s immune system during inhalation of smoke with further increase in reaction to viruses.
Our results demonstrate statistically significant relationship between incidence to Hodgkin lymphoma and number of fires both in FEFD for the age group “children/teens 0–14 years” and in Khabarovskij Kraj for young children up to 4 years (with the threshold α < 0.05). We speculate, that impact of wildfire to development of Hodgkin lymphomas is related to respiratory diseases caused by smoke, which weaken immunity with further activation of new or chronic infection by Epstein–Barr virus (EBV). EBV is a I class carcinogen in the World Health Organization category and pediatric Hodgkin lymphoma (for children up to 10 years) is associated with EBV up to 80% [28].
Leukemia is seen by some authors as “preventable pathology” [29] because observations confirm an increase in leukemia risk with prenatal, in utero, and postnatal exposure to tobacco smoke or automobile gases in young children [30] Our analysis demonstrated a broad range of negative impacts of wildfire smoke on leukemia risk in the prenatal period for young children 0–4 year, so to children/teens 0–14 and entire population leukemia with a variety of time lags.
We found on example of young children up to 4 years in Khabarovsk City/Khabarovskij Kraj that incidence to benign tumors has stronger correlation with number of wildfires in comparison to incidence to malignant tumors. It is known that benign tumors considerably outnumber malignant tumors, but only few of benign tumors got transformed to the malignant ones [31]. It would be useful to extent to the entire territory of FEFD comparison of relationships CI to NF with relationships of incidence to benign tumors to NF in order to study a role of wildfires to evolution of tumors in humans.
CONCLUSIONS
The following conclusions were drawn: 1) Fluctuations in the incidence of hematopoietic, lymphoid, vascular, and soft tissue neoplasms, as well as CNS tumors in young children 0–4 years, children/teens 0-14 years and the entire population of FEFD to some extent are related to wildfires, described by normalized and weighted by population number NF as a proxy. 2) The most sensitive age group to the impact of NF on CI in FEFD is the “entire population” group. 3) Development of different types of neoplasia has different time lags and different relative strengths of statistical dependence from wildfires. Three blood cancers (leukemia, non-Hodgkin lymphoma, and Hodgkin lymphoma) had the strongest associations with wildfires, from the five analyzed types of cancers common for the age cohort and two age population groups. They also have the widest range of time lag, for example, from 2 years of prenatal time lag in the “young children up to 4 years” age cohort to 0–3 years lag for the entire population for “leukemia” type. 4) Statistically significant relationships between NF and CIs were found in spatial regions, which included climatically homogenous AUs of FEFD. Largest number of these relationships are observed for geographical domains with a large number of population. Statistically significant results on relationship of leukemia and non-Hodgkin lymphomas incidence with number of fires for the age group “entire population” and on relationship of Hodgkin lymphomas incidence with number of fires for the age group “children/teens 0–14 years” with time lags 0–3 years can be applied in practice. Influence of fires can be accounted during diagnostic of these diseases as large fire years are well known for the Russian Far East. Further studies should include an analysis of a) environmental, demographic, socio-economic and logistic determinants of spatial and temporal patterns of cancer incidence in FEFD; b) influence of seasonality on statistical relationships between cancer incidence and number of fires; c) influence of number of fires to ratio of benign and malignant tumors. Open is also a question about spatial transferability of our approaches of analysis of relationships between cancer incidence and number of fires to other geographical areas prone to wildfires in Russia (Siberian and Ural Federal districts) and in bordering countries (Kazakhstan and China).