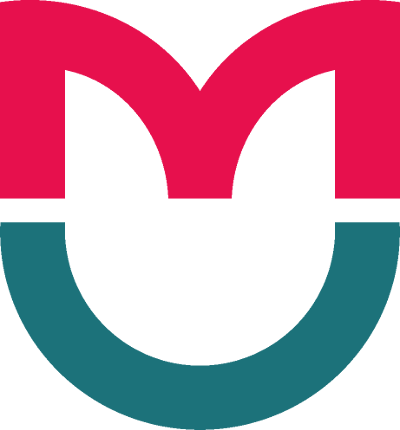
ORIGINAL RESEARCH
Noninvasive preimplantation genetic testing for aneuploidy
Kulakov National Medical Research Center for Obstetrics, Gynecology and Perinatology, Moscow, Russia
Correspondence should be addressed: Olga I. Lisitsyna
Akademika Oparina, 4, Moscow, 117997, Russia; ur.xobni@avokyzay_o
Funding: the study was supported by the Russian Foundation for Basic Research under research project №23-25-00346.
Author contribution: Makarova NP, Lisitsyna OI, Dolgushina NV — study concept and design; Lisitsyna OI, Makarova NP, Dolgushina NV, Syrkasheva AG, Ekimov AN — manuscript writing and editing; Lisitsyna OI — statistical processing of the results; Goryainova EG, Makarova NP — biomaterial collection; Ekimov AN, Atapina EE — laboratory phase; Dolgushina NV, Trofimov DYu — publication approval.
Compliance with ethical standards: the study was approved by the Ethics Committee of the Kulakov National Medical Research Center for Obstetrics, Gynecology and Perinatology (protocol № 10 of 28 October 2021). The patients submitted the informed consent to study participation.
Preimplantation genetic testing (PGT) was introduced into clinical practice in the late 1980s (it was previously referred to as preimplantation genetic diagnosis (PGD) or preimplantation genetic screening). The first PGD programs were aimed to avoid the X-linked inheritance. The range of detecting possible genetic disorders expanded significantly with subsequent development of the embryological and laboratory phase technologies, and the new goals of the methods were both preventing genetic disorders and improving the chances of giving birth to a healthy child in difficult categories of the assisted reproductive technology (ART) patients [1].
Currently, preimplantation genetic testing for aneuploidy (PGT-A) by next generation sequencing (NGS) with trophectoderm (TE) biopsy has become the most effective method that is more and more widely used with the development of the capabilities of embryological and genetic laboratories. Numerous studies demonstrate high sensitivity and specificity of this approach, however, the need for invasive interventions still represents one of its drawbacks [1, 2].
Embryonic mosaicism and concordance between the embryo’s TE chromosomal pattern and its inner cell mass (ICM) are the other issues that attract the researchers’ attention [3, 4]. Despite the fact that biopsy of several cells makes it possible to partially overcome the above shortage, it is impossible to completely eliminate the risk of rejection of the embryo, the transfer of which into the uterine cavity can result in giving birth to a healthy baby.
Noninvasive analysis of cell-free DNA in spent culture medium (SCM), in which the embryo has developed, is a new promising PGT-A technology. Certain conditions of the embryo culture and medium sample collection are required to obtain adequate results (to increase the DNA concentration and reduce possible contamination), however, no invasive intervention is needed [5–7]. A number of researchers believe that noninvasive preimplantation genetic testing for aneuploidy (niPGT-A) is a more helpful PGT-A method, particularly because of the fact that, according to the literature, cell-free DNA contained in SCM originates not only from the TE cells, but also from the ICM [8, 9]. Other authors, on the contrary, argue that it is unrepresentative, since the issue of the true origin of cell-free DNA contained in SCM is yet to be resolved [10, 11]. Anyway, this approach is extensively studied by the researchers and can probably be used in clinical practice.
The study was aimed to assess the efficiency of niPGT-A. For that we conducted re-analysis of blastocysts, that were considered to be aneuploid based on PGT-A with TE biopsy, by niPGT-A.
METHODS
Embryological phase was implemented at the B.V. Leonov Department of Assisted Technologies in Infertility Treatment; PGT-A and niPGT-A were performed in the Institute of Reproductive Genetics.
The study involved 11 embryos from seven couples. All blastocysts were considered to be aneuploid based on previous PGT-A with TE biopsy. The embryos were obtained in ART cycles with PGT-A by NGS in April–September 2020.
Embryo culture, TE biopsy and PGT–A
Fertilization of oocytes was performed by intracytoplasmic sperm injection (ICSI), after that the fertilized cells were transferred to the Continuous Single Culture Complete (CSCM) medium (IrvineScientific; USA). All embryo culture steps and morphological assessment of blastocysts were performed in accordance with the previously reported method [12]. On day 5–6 after fertilization, TE cell biopsy was performed in embryos, the quality of which was considered to be excellent or good according to morphological criteria. Borosilicate glass needles were used for biopsy. After biopsy the embryos were subjected to cryopreservation by vitrification in accordance with the instructions of the culture media manufacturer. The cells obtained were transported to the laboratory in the Eppendorf tubes containing the lysis buffer and stored at a temperature of –20 °C until subjected to further analysis. PGT-A was performed by next generation sequencing (NGS) using the Illumina platform (Illumina; USA) in accordance with the manufacturer's protocol. The results obtained were processed using the SeqVario software (DNA-Technology; Russia).
Embryo thawing and culture, spent culture medium collection
The donated embryos were thawed in the Kitazato media (Kitazato; Japan) in accordance with the manufacturer's protocol. After that blastocysts were individually cultured in 10 µL microdroplets of the CSCM medium for 9 h. A drop of the culture medium (1 sample) kept in the same culture conditions, but containing no embryo, was used as a negative control. All spent culture medium was collected in the Eppendorf tubes and transferred to the laboratory, where the tubes were stored at a temperature of –20 °C for 14 days until used for further analysis.
Noninvasive preimplantation genetic testing for aneuploidy
NiPGT-A was performed using the NICSInst kits (Yicon Genomics; China) in accordance with the manufacturer's instructions. The next generation sequencing was carried out in the NextSeq unit (Illumina; USA). The results obtained were analyzed using the original algorithms and software tools developed by the Center for PGT-A by SeqVario NGS.
Analysis of the results
Statistical analysis was performed using the Jamovi software package (freely distributed statistical software package). The results obtained were tested for concordance with the earlier reported PGT-A data. The full chromosome ploidy concordance rate (based on the PGT-A and niPGT-A data) was considered to be a primary endpoint of the study. The diagnostic concordance rate (euploid/aneuploid) of the embryos based on the PGT-A and niPGT-A data was a secondary endpoint. Binary categorical data were specified as absolute numbers N and the percentage of the total value for group P in the N format (P%). Binomial test was used to determine statistical significance of full and clinical concordance of the results. The significance level (p) was set at 0.05.
RESULTS
A total of 12 SCM samples were analyzed: 11 samples, in which the embryos were cultures, and one control sample. All samples of the study group successfully went through wholegenome amplification and analysis by NGS. No DNA was detected in the control sample. The PGT-A and niPGT-A results along with the concordance of these results for the studied embryos are provided in tab. 1.
It is noteworthy that chaotic niPGT-A results not allowing the karyotype diagnosis were obtained in one case (9.1%) out of 11 (fig. 1).
We failed to detect aneuploidy in an embryo based on the niPGT-A data in one case out of 10 (10%) due to high noise level, that is why the result was considered to be “euploid” (fig. 2).
Full concordance of the results was obtained in seven cases out of 10 (70%) (fig. 3). Concordance of the results based on sex chromosomes was determined in nine cases out of 10 (90%). Clinical concordance of the results was found in nine cases out of 10 (90%).
Comparison of full and clinical concordance between the PGT-A and niPGT-A results is provided in tab. 2. Binomial test revealed no significant differences in the rate of full concordance between the PGT-A and niPGT-A results (p = 0.344), however, a significant result was obtained for diagnostic concordance between the PGT-A and niPGT-A data (p = 0.021).
When assessing the results in accordance with the intention to study, i.e. when assessing all 11 embryos that were included in the study in practical terms, the results obtained meant that in 81.8% of cases the clinical decision about the possibility of embryo transfer in the uterine cavity would remain unchanged. In 9.1% of cases, the clinical decision about the possibility of embryo transfer made based on the niPGT-A data only would be different (an euploid embryo was recommended for transfer). It was impossible to make a clinical decision about the possibility of embryo transfer in 9.1% of cases.
DISCUSSION
The study planned as a pilot project was limited by small sample size, availability of aneuploid embryos only (based on the PGT-A data), no analysis of the ICM chromosomal composition in the studied blastocysts. Nevertheless, the study results showed that cell-free DNA (cfDNA) was detected in SCM in 100% of cases, a high proportion of results appropriate for clinical interpretation (90.9%) was also reported.
In the majority of studies conducted so far the data of PGT-A with TE biopsy have been considered as reference data and compared with the niPGT-A results. The concordance rate of the results in these studies is 33.3–89.1% [5, 8, 13–17]. However, it should be noted that the above papers more often described the protocol for niPGT-A in a fresh cycle. Our study involved the protocol for thawed embryos, and the concordance rate was consistent with the data of the above studies (full concordance in 70% of cases, diagnostic concordance in 90% of cases).
In other studies the whole embryo was considered as a reference, and SCM was collected after the post-thawing embryo culture. The concordance rate of the niPGT-A and PGT-A results for the whole embryo in such studies varied between 32.2–89.9%. Xu et al. assessed SCM of the thawed D3 embryos after culturing to 5 days. Among 42 embryos, assessment of 66.7% showed full concordance of the results [18]. Yin et al. assessed SCM of 75 thawed D5–D6 blastocysts after culturing for 24 h. The diagnostic concordance rate of the results was 89.8%, while full concordance rate was 32.2% [19]. Huang et al. managed to get information about SCM of 48 D5–D6 embryos out of 52 after thawing and culturing for 24 h. Full concordance rate of the results was 85.4% [9]. Shitara et al. reported full concordance rate of 56.3% when assessing SCM after thawing and culturing 5D embryos for 24 h and 6D embryos for 3 h [20]. Xu et al. assessed 35 thawed 3D and 5D embryos. The minimum incubation time was 24 h.
A total of 88.6% SCM samples successfully went through whole-genome amplification. Full concordance rate of the ICM niPGT-A and PGT-A results was 58.3% (14/24) [21].
It should be noted that the culture medium drop volume in these studies involving culturing thawed embryos varied between 10–30 µL, while the test sample volume was 3.5–25 µL. Incubation time was at least 24 h in the majority of cases. Our study demonstrated successful detection and analysis of cfDNA in SCM when culturing thawed blastocysts for 9 h in the 10 µL drops, thereby showing the possibility of obtaining adequate results after culturing thawed embryos within a shorter time period.
Of particular interest is the study of additional options to use niPGT-A in clinical practice. Noteworthy is the paper, the authors of which performed niPGT-A for mosaic embryos based on the results of previous PGT-A with TE biopsy. They thawed and re-cultured 41 mosaic embryos for 14–18 h, then performed PGT-A with TE biopsy of the whole embryo and SCM niPGT-A. The results of ICM assessment showed that 84.4% of embryos (35/41) had normal chromosome pattern. The niPGT-A data were concordant with the results of PGT-A based on the whole embryo biopsy in 74.4% of cases [22]. In the paper published the authors reported retrospective data on the transfer of mosaic embryos in 60 couples having no euploid embryos. Clinical pregnancy was later diagnosed in 30 patients. Thus, the researchers assumed that additional use of niPGT-A in such clinical cases had some possible benefits.
In our study we also obtained one “euploid” niPGT-A result for aneuploid embryo (karyotype (21) × 1.5) based on the data of previous PGT-A with TE biopsy. Furthermore, no abnormality of the 21st pair of chromosomes was detected (fig. 2). Given the above, it is reasonable to collect SCM in the cycles with PGT-A in order to explore the possibility of additional niPGT-A use for more correct diagnosis in complicated or questionable cases (if necessary).
Also of interest is the paper published in 2022 focused on assessing the chances of the clinical use of niPGT-A in the thawed embryo transfer ART cycles [23]. The embryos were thawed and cultured in the 20 µL drops for 6 h in all cases until transferred into the uterine cavity. The authors retrospectively assessed the outcomes of thawed embryo transfer in 210 patients based on the niPGT-A results. The rates of clinical pregnancy, ongoing pregnancy and live birth were significantly higher in embryos that were considered to be euploid based on niPGT-A compared to aneuploid embryos (56.2% vs. 29.4%). However, no differences in the above reproductive outcomes between “euploid” and “chaotic” embryos were revealed based on the niPGT-A data (56.2% vs. 60.4%). The percentage of aneuploid embryos was significantly higher among the embryos that were considered to be of low or medium quality based on morphological features compared to the good quality embryos (46%, 34.6% and 21.5%, respectively; p = 0.013). The researchers noted a possible advantage of using a combination approach (morphological assessment in combination with niPGT-A) to selection of the most promising embryo in terms of transfer into the uterine cavity. Furthermore, the authors proposed to rank the embryos in order of decreasing priority for embryo transfer in the following way: 1) good quality euploid embryos; 2) good quality “chaotic” embryos; 3) euploid embryos of medium quality based on morphological features; 4) “chaotic” embryos of medium quality based on morphological features.
It should be emphasized that other researchers also recommend to interpret chaotic niPGT-A results with caution, since such results are more likely to reflect the biomaterial storage conditions and DNA degradation processes than the embryos’ chromosomal patterns [8, 24]. In our study we have also obtained chaotic niPGT-A results in one case (9.1%) (fig. 1).
Thus, it should be noted that dealing with cfDNA and niPGT-A has some features that should be taken into account when interpreting the results and selecting the most promising embryos for transfer into the uterine cavity. However, the noninvasive nature of the method should be noted as its chief feature. Considering the fact that a number of scientific papers report the data indicative of possible adverse effects of TE biopsy on the course of pregnancy (hypertensive disorders, premature birth, placental disorders) and the health of newborns, it is the study of niPGT-A that seems to be the most relevant [25–27]. However, it should be noted that no such correlations have been revealed in a large number of other studies [28–30].
CONCLUSIONS
Noninvasive preimplantation genetic testing for aneuploidy is a promising new method to assess the embryo’s chromosomal status that does not require biopsy. Further research is necessary to further develop and improve the method, as well as to determine the possibility and indications for the use of method in clinical practice.