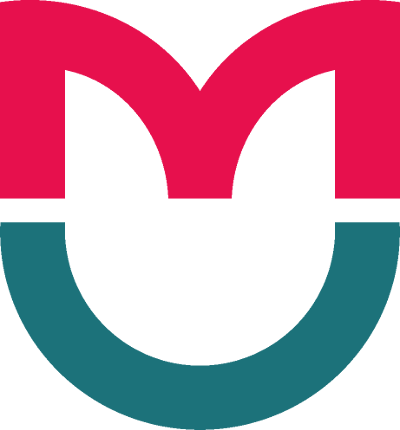
ORIGINAL RESEARCH
Prefrontal cortex transcranial theta-burst stimulation frequency-dependent effects on cognitive functions
Research Center of Neurology, Moscow, Russia
Correspondence should be addressed: Alexandra G. Poydasheva
Volokolamskoye shosse, 80, Moscow, 125310, Russia; ur.ygoloruen@avehsadyop
Funding: the study was supported by the Russian Science Foundation (RSF), grant № 22-25-00078, https://www.rscf.ru/project/22-25-00078/
Author contribution: Suponeva NA, Bakulin IS, Poydasheva AG, Piradov MA — study concept; Suponeva NA, Bakulin IS, Poydasheva AG, Sinitsyn DO, Piradov MA — study planning and design; Bakulin IS, Poydasheva AG, Zabirova AH, Lagoda DYu — research procedure and data acquisition; Bakulin IS, Poydasheva AG, Sinitsyn DO, Zabirova AH — data analysis; all authors — data interpretation; Suponeva NA, Bakulin IS, Poydasheva AG — manuscript draft writing; all authors — manuscript editing.
Compliance with ethical standards: the study was approved by the Ethics Committee of the Research Center of Neurology (protocol 3-7/22 dated 20 April 2022), it was conducted in accordance with the principles of the Declaration of Helsinki; the informed consent was submitted by all study participants.
Non-invasive neuromodulation, specifically transcranial magnetic stimulation (TMS), is used both in the study of cognitive function and as a potential method to enhance it in healthy individuals and patients [1–3].
The effect of repetitive TMS is thought to be due to its influence on neuroplasticity mechanisms [4, 5]. It is not confined to the stimulated area and extends over the functionally linked components of neural networks [1]. In recent years, an increasing attention was paid to investigation of patterned TMS protocols, including theta-burst stimulation (TBS), when “bursts” of three stimuli with a specified frequency (usually 30 or 50 Hz) are delivered at a frequency of 3 or 5 Hz. Bursts can be presented continuously for 20 or 40 s (continuous TBS, cTBS) or for 2 s with an interval of 8 s (intermittent TBS, iTBS) [6–7]. TBS is characterized by the sufficiently long neuromodulatory effect duration with short duration of stimulation, which makes the use of TBS in clinical practice promising [8].
The data on the TBS effect on cognitive functions in healthy volunteers are controversial [9]. One of the reasons can be high inter-individual variability in response to stimulation reducing the group effect. Furthermore, are poorly reproducible at the individual level: cognitive function improvement in healthy volunteers in response to the TBS session is observed in 70% of cases, however, it is reproduced only in 37.5% when reassessed on a different day [10].
The development of personalized protocols (particularly, based on stimulation frequency) represents one of the approaches to variability reduction. In addition to selection based on individual cortical rhythm frequencies [11, 12], selection based on the cortical oscillation phase-amplitude coupling (PAC) is a relatively new method.
The most studied variant of PAC is the theta-gamma coupling (TGC), which is important for memory processes. In TGC the amplitude of oscillations in the gamma range (over 30 Hz) is modulated by phase of oscillations in the theta range (4–8 Hz) [13–15]. TGC has been shown to correlate with working memory (WM) test scores and several other cognitive functions in healthy volunteers [16] and its decline has been observed in Alzheimer's disease, moderate cognitive impairment and mental disorders [13, 17].
The results of sporadic studies involving the use of the noninvasive neuromodulation protocols personalized based on the TGC frequency are available. A significantly more prominent effect of one session of personalized protocol on the n-back test results in healthy volunteers compared to standard iTBS (6/30 and 5/50 Hz) when performing assessment 45 min after stimulation was revealed, along with significant differences in the characteristics of the TMS-evoked EEG potentials between the standard and personalized protocols [18]. Considering these data, the study was aimed to assess the effects of the left dorsolateral prefrontal cortex (lDLPFC) personalized stimulation protocol based on TGC on the cognitive test results in healthy volunteers and to compare its efficacy with that of standard protocol and sham stimulation.
METHODS
Prior to inclusion in the study, participants completed a questionnaire to identify contraindications to TMS and a modified version of the Edinburgh Handedness Inventory (EHI) [19]. Medical history of each participant was taken and demographic data were acquired, routine electroencephalography (EEG) with standard functional tests was performed to exclude epileptiform activity (Neuron-Spectr 4/P, Neurosoft LLC; Russia). Inclusion criteria: informed consent; age 18–40 years; laterality index > 40 based on EHI. Non-inclusion criteria: contraindications to MRI/TMS; epileptiform activity on the routine EEG; history of neurological or mental disorders; the use of medications having an effect on the CNS; decompensated chronic somatic disorder. Exclusion criteria: severe adverse events (AEs) revealed during the TMS procedure (TMS-induced epileptic seizure, syncope, etc.); development of somatic, mental or neurological disiases; pregnancy; refusal to participate.
Determining individual theta–gamma coupling frequencies
To determine the TGC frequencies, the 64-channel EEG (10–10 electrode placement system) synchronized with the n-back test (Latin consonants were presented with n = 3, the details of the method are provided below) was recorded. The actiCHamp Plus 64 (BP-100-2511) system (Brain Products GmbH; Germany) was used for EEG recording; synchronization was performed using the Trigger Station (Brain Trends; Italy); paradigm was written in the E-Prime 3.0 software (Psychology Software Tools; USA). Three series of 24 stimuli were presented (6 matching stimuli per session were presented at 3000 ms intervals, the stimulus presentation time was 250 ms. There were breaks for rest between series, the duration of which was determined by volunteers (10–20 s). Training with n = 1 and 2 was conducted before the main test.
EEG preprocessing and analysis was performed in EEGLAB and MATLAB (The MathWorks, Inc.; USA). Preprocessing involved removal of channels with high noise levels; referencing to the average reference; 1–80 Hz band filtering with a 49–51 Hz band-stop filter; removal of intervals with artifactualsignal; artifact removal by independent component analysis. The signals from the Fz and Pz electrodes were analyzed to calculate the phase and amplitude of the TGC, respectively [18]. The averaged signal of all recording electrodes was used as a reference. We analyzed intervals from 250 ms after the end of the stimulus display to the moment of the next stimulus display with a cutting of 750 ms on each side to reduce edge artifacts (69 intervals of 1000 ms each). Wavelet transform was applied with the cycle number of 6 and 4 for phase and amplitude to extract rhythms. The coupling strength was estimated using the mean vector length (MVL) as the most sensitive under the assumption of monophasic coupling. The index normalization procedure was used to eliminate unbalanced phase distribution and large amplitude fluctuations. The TGC comodulograms were plotted for each subject. The frequencies of maximum TGC were used to develop a personalized iTBS protocol.
Theta-burst stimulation protocols
TMS was performed with the MagPro X100 + MagOption stimulation device (Tonika Elektronik A/S; Denmark) in combination with the Localite TMS Navigator system (Localite GmbH; Germany) and the Axilum Robotics TMS-Cobot robotic positioning system (Axilum Robotics; France) using the liquidcooled figure-of-eight coil. For navigated TMS, MRI was performed in the 3D-Т1-MPR (3D T1 multiplanar reconstruction) mode using the MAGNETOM Verio and MAGNETOM Prisma scanners (Siemens Healthcare GmbH; Germany) (voxel size 1.0 × 0.977 × 0.977 mm3, 176 sagittal slices). The target within lDLPFC was defined as a spot located 5 cm anterior to the “hot spot” of the first dorsal interosseous (FDI) muscle cortical representation; the localization was specified based on individual structural MRI data. Electromyography recording of motor evoked responses involved the use of the Ag/Cl electrodes (Neurosoft LLC; Russia) placed on the muscle belly (active electrode) and the tendon (reference electrode). Intensity equal to 75% of individual resting motor threshold determined in accordance with the Rossini-Rothwell protocol for FDI prior to each session was used in the active stimulation protocols.
During the crossover study each subject underwent one stimulation session according to the protocols below with at least 72 hours between sessions. All sessions were conducted within the same time frame (8–14 or 14–17 hours). Protocols were pseudo-randomized by the Latin square method and included the following:
- iTBS-5/50 — standard iTBS protocol (bursts of 3 stimuli at 50 Hz, bursts were applied with a frequency of 5 Hz). One block consisted of 10 bursts, an interval between blocks was 8 s, the total number of stimuli per session was 600;
- iTBS-ind — personalized iTBS protocol (bursts of 3 stimuli, the stimulus frequency corresponded to the gamma rhythm frequency and the frequency of bursts corresponded to the frequency of theta rhythm at maximum TGC determing during the previous step). One block consisted of 10 bursts, an interval between blocks was 8 s, the total number of stimuli per session was as close to 600 as possible for this frequency ratio;
- iTBS-sham — sham stimulation involved the use of special figure-of-eight coil imitating the sounds of stimuli presented, but not inducing stimuli themselves, and local low-intensity electrical stimulation imitating the sensations of magnetic stimulation. The duration and frequency of the sound imitation of stimuli were similar to standard, and the electrical stimulation intensity was determined individually based on the severity of sensations in the area subjected to stimulation.
Cognitive tests
Neuropsychologial testing was performed using PEBL v 2.0 (Psychology Experiment Building Language) [20]. Participants put on the noise-canceling headphones to minimize distractions. The following tests were used: n-back test involving simultaneous presentation of spatial and verbal stimuli; backwards Corsi blocks task; Forward Digit Span; Tower of London.
In the n-back test, the subjects were simultaneously presented with spatial (position of squares) and verbal (Latin consonants) stimuli [21]. The subject had to determine whether the current stimulus matched the one presented n steps before and to press the key when there was a match. The accuracy of performance was assessed using the d’ sensitivity index [22]. It was calculated based on the number of correct keystrokes in response to to a matching stimulus (hits) and and the number of false keystrokes in response to a mismatching stimulus (false alarms). The number of hits was normalized to the total number of matching stimuli (6 for the version of the task used), and the number of false alarms was normalized to the total number of mismatching stimuli (16 and 17 for n-back test with n = 2 and n = 3, respectively). Z transformation was applied to each normalized value, and d’ was calculated as a difference of Z-values for hits and false alarms. Considering the simultaneous presentation of verbal and spatial stimuli, the total d’ value for two types of stimuli with each n was also determined. The results obtained with n = 2 and n = 3 were analyzed. Training involving separate presentation of verbal stimuli with n = 1 and 2, spatial stimuli with n = 1, and the combination of two types of stimuli with n = 1 was performed before each n-back test.
In the Corsi blocks task, one had to memorize the order, in which squares were presented, and reproduce it in reverse order. The maximum length of the correctly recalled sequence was estimated.
In the Digit Span test, the subject was presented a numerical sequence, which he/she had to reproduce in direct order, starting from the sequence of 5 digits, with the step of 1 digit, up to a maximum length of 10 digits. The maximum length of numerical sequence the subject could correctly recall or the total number of correctly recalled sequences (after the maximum length of 10 digits was achieved) was estimated.
In the Tower of London test, the subject was offered to reproduce the specified sequence of colored disks by moving the disks. The time of task performance was recorded.
The test scores were measured at three time points: Т0 — before stimulation, Т1 — immediately after the end of stimulation, Т2 —1 h after the end of stimulation. The effects of stimulation at Т1 and Т2 were estimated as the difference between the values at each of these points and the initial value at Т0.
Statistical data processing
Statistical analysis was performed using the IBM SPSS Statistics software package (v.23) (IBM, SPSS Inc.; USA). Estimation of individual effects of each protocol at Т1 and Т2 compared to Т0 as well as comparison of effects between different protocols was peformed using the Friedman test. The Wilcoxon test with Bonferroni correction was used to identify significant differences for pairwise comparisons. The significance threshold was set as p = 0.05.
Assessment of the stimulation protocol safety and tolerability
All participants completed the standardized questionnaire for assessment of AEs during the TMS session immediately after stimulation and within 24 h after stimulation, before each subsequent stimulation session.
RESULTS
A total of 25 individuals were screened, among them four had contraindications to TMS (three individuals used drugs affecting the CNS, one had somatic disorder). Among the included volunteers five failed to complete the study (four for logistical reasons, one due to poor tolerability of TMS). The data of 16 volunteers were included in the final analysis (seven males, average age 29.6 years).
Individual theta–gamma coupling frequencies
The following TGC frequencies used for individual protocols were obtained based on the analysis of the EEG data with paradigm (tab. 1). An example of individual comodulogram is provided in figure.
Safety and tolerability
The data of 50 sessions were analyzed (16 iTBS-ind, 17 iTBS5/50, 17 iTBS-sham). No severe AEs were reported. One volunteer refused to continue participating after the second session of stimulation (iTBS-5/50) due to the development of mild headache (3 points on the Numeric Pain Scale, NPS) and difficulty concentrating.
Mild AEs were reported in 29 sessions (78%) (tab. 2). Mild to moderate pain in the area of stimulation was the most common AE reported during TMS (12 sessions, 24%; 2–5 points on NPS). more intense pain (7–8 points on NPS) was reported during the iTBS-ind stimulation in one case and during the iTBS-sham in one case. Headache within 24 h after stimulation also had low intensity (2–3 points on NPS).
Cognitive effects
Analysis of effects of distinct protocols
When assessing the effects of distinct protocols using the Friedman test assessing differences at three time points), significant differences were revealed after the iTBS-5/50 protocol for the n-back tests with spatial stimuli (n = 3) (p = 0.013), Corsi blocks (p = 0.044) and Tower of London (p = 0.015), as well as after the iTBS-ind and iTBS-sham protocols for the Tower of London test (p = 0.02 and p = 0.006, respectively).
When performing pairwise comparison using the Wilcoxon test, significant differences were reported for the iTBS-5/50 protocol in the n-back test with spatial stimuli (n = 3) between measurements at Т2 and Т0 (pcorr = 0.018, Bonferroni correction for multiple comparison) and in the Tower of London test between measurements at Т2 and Т1 (pcorr = 0.039); comparisons of other tests turned out to be non-significant. As for protocols iTBS-ind and iTBS-sham, significant differences were reported in the Tower of London test between measurements at Т2 and Т0 (pcorr = 0.045 and pcorr = 0.003, respectively) (tab. 3).
Comparison of effects between protocols
When comparing protocols, significant differences were reported in the n-back test with spatial stimuli (n = 3) for blocks task for differences between Т0 and Т2 and between Т1 and Т2 (tab. 4). Pairwise comparison involving the use of the Wilcoxon test revealed significant differences in the n-back test with spatial stimuli (n = 3) between protocols iTBS-5/50 and iTBS-sham (the effects of the iTBS-5/50 was larger) (pcorr = 0.039), in the Corsi blocks task between points Т2 and Т1 for protocols iTBS-5/50 and iTBS-ind, as well as iTBS-5/50 and iTBS-sham (the effects of the iTBS-5/50 protocol was smaller compared to other protocols; pcorr = 0.038 and 0.048, respectively) (tab. 5).
DISCUSSION
During the study we developed an lDLPFC iTBS personalized protocol based on individual TGC frequencies, gained experience of its application on healthy volunteers and showed a beneficial safety and tolerability profile. No significant differences from the standard protocol or sham stimulation were reported in the majority of tests. Significant effect was reported only in the Tower of London test for assessment of executive functioning. It was shown that standard protocol was effective for the spatial WM and divided attention test (n-back test with n = 3), and the change was significantly larger compared to sham stimulation. On the other hand, the simpler spatial WM test (Corsi blocks) revealed significantly worse execution after this protocol showing significant differences from sham stimulation and personalized protocol.
In contrast to the earlier reported data, we failed to find benefits of the effects of personalized protocol compared to the standard protocol, there also were no differences from sham stimulation [18]. The observed decrease in task completion time in the Tower of London test may be due to learning, since the same statistically significant effect was observed during sham stimulation. However, no significant differences between protocols were revealed in this test. Perhaps, the lack of the personalized protocol effect results from the destructive TMSinduced activity interference with individual cortical rhythms. This assumption is in line with the results of studies focused on the use of transcranial alternating current stimulation (tACS), in which the effect was dependent on the theta cycle phase, during which the gamma frequency current was applied [23, 24]. When using TMS, control of the theta bursts’ synchronization with endogenous theta rhythm is possible only with the use of the “closed-loop” systems with sumiltaneous EEG-TMS recording and synchronization of stimuli with the peaks of individual theta rhythm [25], however, the protocol efficacy and feasibility need to be studied.
The reported spatial WM improvement in response to one session of standard iTBS protocol is compliant with the results of some other studies [26, 27]. The use of the n-back test variant with simultaneously presented verbal and spatial stimuli assessing both WM characteristics and divided attention is a peculiarity of our study. No significant differences were observed when evaluating the test with verbal stimuli and the total score, so it is unlikely that the observed effect is due to a change in task strategy. The standard protocol had an oppositely directed effect on the other spatial WM test — Corsi blocks, i.e. a decrease in the number of memorized stimuli, although it was non-significant. The possible explanation could be that the lDLPFC stimulation affected mainly the processes of information processing in the WM or divided attention important for the n-back test execution, while the process of information maintenance was more important for the Corsi blocks task. Such multidirectional effects of protocol on two important WM components, information maintenance and processing, agrees well with the concept of the zero-sum game, according to which improvement of one function in response to stimulation is accompanied by deterioration of the other one and eventually leads to a zero result [28].
The emergence of significant differences in testing only after the standard iTBS protocol can be explained by the fact that stimulation with a frequency of 5 and 50 Hz is most effective for induction of the long-term potentiation, as has been earlier shown in animal models [6]. An alternative explanation can be based on the assumption that the WM capacity is determined by the ratio of gamma/theta frequencies. In this case stimulation with a frequency lower than individual theta rhythm leads to the increase in the WM capacity, which has been confirmed by studies involving the use of tACS [29, 30]. A similar approach can be realized using iTBS protocols.
All detected effects were noted when performing delayed measurements (an hour after the beginning of stimulation), while measurements performed immediately after stimulation revealed no significant differences for any of the protocols. The duration of the effect is consistent with the results obtained earlier, according to which the effect on cognitive functions is absent when measured immediately after stimulation, but is detected in repeated measurements in the delayed period (60 min in our study, 20 and 40 min — according to the literature) [26]. It is reasonable to estimate the stimulation effects not only immediately after the end of stimulation, but also throughout the intended effect duration.
Small sample size can be considered the study limitation, however, this power is sufficient to detect "large" effects. Furthermore, variability of individual TGC and the issues related to selection of algorithm for individual TGC calculation represent a significant limitation. The lack of significant effect on the results of some tests (such as n-back test with n = 2; Digit Span, Corsi blocks) can also be due to their low complexity and the “ceiling effect”. One more limitation is the crossover design that can result in learning effect between sessions. However, the possible bias due to learning was minimized by the Latin square method. Furthermore, volunteers undervent several training tests before the beginning of stimulation sessions.
CONCLUSIONS
Thus, we have demonstrated safety and efficacy of the lDLPFC personalized theta-burst stimulation based on individual TGC frequencies, but the effect on cognitive test scores could not be confirmed. Standard iTBS protocol turned out to show a significant effect different from sham stimulation. In this regard, further investigation of this protocol, for example, in patients with cognitive impairment, can be promising. Considering the results obtained, it seems reasonable to test the alternative hypotheses when conducting further research (for example, the hypothesis of individual stimulation with frequencies lower than the measured TGC frequencies or stimulation phase synchronized with theta rhythm). Furthermore, it is necessary to further investigate optimal TGC calculation algorithms to improve measurement accuracy and reproducibility.