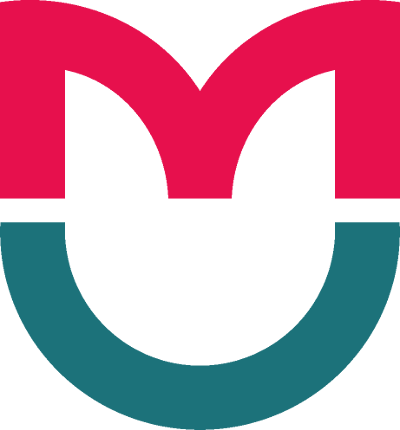
This article is an open access article distributed under the terms and conditions of the Creative Commons Attribution license (CC BY).
OPINION
Potential of non-traditional cell cultures for production of biotherapeutic proteins
1 Shemyakin–Ovchinnikov Institute of Bioorganic Chemistry RAS, Moscow, Russia
2 Moscow Institute of Physics and Technology (MIPT), Dolgoprudny, Russia
3 Pirogov Russian National Research Medical University, Moscow, Russia
Correspondence should be addressed: Zinaida Mikhailovna Osipova
Miklukho-Maklaya, 16/10, Moscow, 117997; ur.hcbi@avoksakz
Funding: the work was supported by the Russian Science Foundation grant No. 21-74-10152, https://rscf.ru/project/21-74-10152/
Acknowledgements: the authors thank A.D. Barykin from the Department of Bioorganic Chemistry of the Institute of Bioorganic Chemistry of the Russian Academy of Sciences for the fruitful discussion of the idea of this publication.
Author contribution: Dobronos MA — literature search and analysis, manuscript authoring; Osipova ZM — project management, manuscript editing; Markina NM — manuscript idea, literature search and analysis, manuscript authoring.
Today, one of the key tasks before the pharmaceutical industry is to increase the efficacy of production of biotherapeutic drugs. Depending on the desired composition of the drug, the stages that may present hindering obstacles are the search for a natural source thereof or the development of its artificial analogue, boosting production by the source, or optimization of purification (removal of impurities and ineffective forms) [1, 2]. We would like to present the potential of non-traditional cell cultures as a key part of the solutions to such problems.
Cell cultures are most common in the production of biotherapeutic protein preparations, with monoclonal antibodies being the most significant thereof. The flagship culture is that of Chinese hamster ovary cells (CHO): it is easy to cultivate and grows rapidly, guarantees correct translation, folding and posttranslational modification of the recombinant protein, and releases large amounts of the product into the culture medium, yielding the largest amounts of the target drug among all mammalian cell cultures [3]. The drawbacks of all mammalian cell cultures, including CHO, are high cost and need for special working conditions and equipment, as well as susceptibility to metabolic burden. When the output of the recombinant protein reaches a certain level, this burden prevents standard production boosting technologies from working, including those that involve increasing the number of copies of the recombinant gene, using stronger regulatory sequences, etc. [4]. This happens because recombinant processes begin to compete for resources with the host cell's viability maintenance processes; there are about 8–10 of these in total, including the processes of transcription, translation, post-translational modifications, and protein export [5]. Various metabolism balancing techniques are used to counter the negative effects of metabolic burden, but this is a very labor-intensive process, since it is necessary to identify all the limiting stages [6] and choose the method to overcome them without compromising the overall viability of the producing cell [7, 8]. However, even successful metabolism balancing may not yield a significant boost in recombinant protein production, since in the case of some biotherapeutic proteins, the process is so laborious for a mammalian host cell that all attempts to optimize it are limited by the physiological capabilities of that cell. For example, recombinant production of the blood coagulation factor VIII (F8) in the CHO culture has the approximate "energy cost" of about 10,000 ATP molecules per a functional F8 molecule [5].
As an alternative, protein preparations can be produced in hosts whose physiological resources are initially higher than those of mammalian cells; such hosts are cells from other animal species or orthogonal cellular systems, like plant cell cultures (table). One of the main advantages of plant-based biotherapeutic compounds is their safety: they cannot be infected with human pathogens, produce no endotoxins, and have reduced immunogenicity, which improves drug tolerance and minimizes side effects. For example, taliglucerase alpha (β-D-glucosyl-N-acylsphingosinglucohydrolase) produced in transgenic carrot cells for treatment of Gaucher disease type 1 has shown to not trigger any evident side effects associated with N-glycan residues during clinical trials. Moreover, no antibodies to this drug have been detected [9]. In addition, biological preparations produced in plant cell cultures can be administered orally without purification or with minimal purification. Plant cell walls can protect biological products from enzymatic degradation in the gastrointestinal tract, as well as facilitate the delivery of these drugs to the intestine lymphoid tissue in the active form. Clinical trials have shown that production of oral biopharmaceuticals from edible plant tissues is feasible [10].
Plant cell cultures allow achieving a high level of expression of multiprotein complexes that require complex folding and assembly processes, which is also an important aspect in the context of their use for the purpose. Strategies involving construction of a single vector with a set of recombinant genes and joint biosynthesis of recombinant proteins together with chaperones of the same origin can help increase the output of such complexes [11]. In addition, introduction of an exogenous signal sequence directing the protein along a specific secretory pathway can increase the yield of small proteins weighing less than 30 kDa. We believe that optimization of the fermentation process, including continuous or semi-continuous fermentation, is a universal method of increasing protein output from both plant and insect cell cultures.
Insect cell lines Spodoptera frugiperda Sf21, Sf9 and Trichoplusia ni BTI- 5B1-4 (High Five), adherent nonpermissive cell cultures obtained from ovarian tissues of the respective insects, are also widely in production of biotherapeutic proteins in baculovirus expression systems [12]. Insect cell cultures offer similar mechanisms of post-translational modification of proteins, which makes them a cost-effective and scalable tool for the production of vaccine antigens and virus-like particles [13]. Besides, engineered baculoviruses with mammalian promoters (BacMam) possess a significant potential as vectors for gene delivery to mammalian cells [14]. The glycosylation pattern in these expression systems differs slightly from that of humans, but can be humanised through parallel expression of mammalian glycotransferases and the removal of insect-specific alpha-1,3-fucosylated glycans, which can cause allergies in people. Antigen proteins that are core of candidate vaccines against COVID-19 [15–17] and malaria [18] are produced in insect cell cultures.
CRISPR technology can help significantly accelerate the production of stably expressing glycoengineered insect lines. It has been shown that CRISPR can be used to knock out genes in the Drosophila and Bombyx cell lines, as well as to knock out the N-acetylglucosaminidase gene in the S2 cell line, which triggers exponential growth of the number of GlcNAc terminal residues in recombinant human erythropoietin [19]. Another promising direction is modification of the Sf9 and High Five cell lines, e.g., multiple duplication of the mammalian glycotransferase genes, can secure an even higher level of protein expression with correct glycosylation and folding.
Worm cell lines are a viable alternative for a number of biomedical applications. For example, embryonic C. elegans cell cultures are used to study the processes of cell differentiation, morphogenesis, and gene expression, opening up a wide range of previously experimentally inaccessible opportunities [20]. Somatic cells from various tissues of C. elegans (neurons, muscle cells, hypodermic and intestinal cells, etc.) can be cultured for investigation of tissue-specific interactions and signaling pathways [21]. Cell culture of Schistosoma mansoni, a parasitic flatworm, which can be continuously cultivated for 6 months [22], may also become an interesting tool for studying the parasite-host interactions and anthelmintic drugs testing. Many species of marine worms are sources of biologically active compounds, including peptides with antimicrobial, antiinflammatory, immunomodulatory, antioxidant, and antihypoxic effects [23]. The development and optimization of the technology to isolate and cultivate worm cells (long-term cultivation) could boost screening and subsequent effective development of such biologically active substances.
CONCLUSION
Thus, the use of nontraditional cell cultures is a promising way to increase the efficiency of the production of biotherapeutic drugs. Several features of expression mechanisms in alternative cultures can minimize side effects and improve tolerability of the resulting protein preparations. Moreover, alternative producing organisms also help to circumvent the limitations associated with the increased metabolic burden in mammalian cell cultures. This enables further development and production of more effective and affordable biotherapeutic drugs, contributing to the overall progress in the fields of pharmaceuticals and medicine.