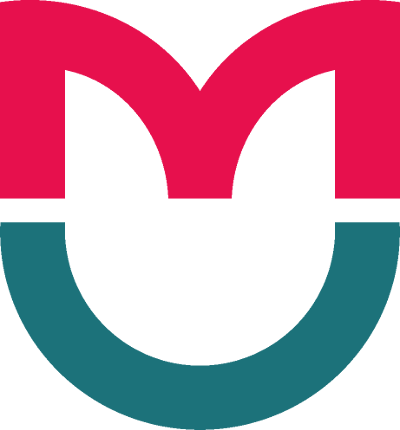
OPINION
Potential of non-traditional cell cultures for production of biotherapeutic proteins
1 Shemyakin–Ovchinnikov Institute of Bioorganic Chemistry RAS, Moscow, Russia
2 Moscow Institute of Physics and Technology (MIPT), Dolgoprudny, Russia
3 Pirogov Russian National Research Medical University, Moscow, Russia
Correspondence should be addressed: Zinaida Mikhailovna Osipova
Miklukho-Maklaya, 16/10, Moscow, 117997; ur.hcbi@avoksakz
Funding: the work was supported by the Russian Science Foundation grant No. 21-74-10152, https://rscf.ru/project/21-74-10152/
Acknowledgements: the authors thank A.D. Barykin from the Department of Bioorganic Chemistry of the Institute of Bioorganic Chemistry of the Russian Academy of Sciences for the fruitful discussion of the idea of this publication.
Author contribution: Dobronos MA — literature search and analysis, manuscript authoring; Osipova ZM — project management, manuscript editing; Markina NM — manuscript idea, literature search and analysis, manuscript authoring.
- Daboussi F, Lindley ND. Challenges to Ensure a Better Translation of Metabolic Engineering for Industrial Applications. In: Selvarajoo K, editor. Comput Biol Mach Learn Metab Eng Synth Biol., New York, NY: Springer US; 2023: 1–20. Available from: https://doi.org/10.1007/978-1-0716-2617-7_1.
- Rudge SR, Ladisch MR. Industrial Challenges of Recombinant Proteins. In: Silva AC, Moreira JN, Lobo JMS, Almeida H, editors. Curr Appl Pharm Biotechnol, Cham: Springer International Publishing; 2020: p. 1–22. Available from: https://doi.org/10.1007/10_2019_120.
- Meleady P, Doolan P, Henry M, Barron N, Keenan J, O’Sullivan F, et al. Sustained productivity in recombinant Chinese Hamster Ovary (CHO) cell lines: proteome analysis of the molecular basis for a process-related phenotype. BMC Biotechnol. 2011; 11: 78. Available from: https://doi.org/10.1186/1472-6750-11-78.
- Frei T, Cella F, Tedeschi F, Gutiérrez J, Stan G-B, Khammash M, et al. Characterization and mitigation of gene expression burden in mammalian cells. Nat Commun. 2020; 11: 4641. Available from: https://doi.org/10.1038/s41467-020-18392-x.
- Gutierrez JM, Feizi A, Li S, Kallehauge TB, Hefzi H, Grav LM, et al. Genome-scale reconstructions of the mammalian secretory pathway predict metabolic costs and limitations of protein secretion. Nat Commun. 2020; 11: 68. Available from: https://doi.org/10.1038/s41467-019-13867-y.
- Kaste JAM, Shachar-Hill Y. Model validation and selection in metabolic flux analysis and flux balance analysis. Biotechnol Prog. 2024; 40: e3413. Available from: https://doi.org/10.1002/btpr.3413.
- Eisenhut P, Marx N, Borsi G, Papež M, Ruggeri C, Baumann M, et al. Manipulating gene expression levels in mammalian cell factories: An outline of synthetic molecular toolboxes to achieve multiplexed control. New Biotechnol. 2024; 79: 1–19. Available from: https://doi.org/10.1016/j.nbt.2023.11.003.
- Kol S, Ley D, Wulff T, Decker M, Arnsdorf J, Schoffelen S, et al. Multiplex secretome engineering enhances recombinant protein production and purity. Nat Commun. 2020; 11: 1908. Available from: https://doi.org/10.1038/s41467-020-15866-w.
- Aviezer D, Brill-Almon E, Shaaltiel Y, Hashmueli S, Bartfeld D, Mizrachi S, et al. A Plant-Derived Recombinant Human Glucocerebrosidase Enzyme — A Preclinical and Phase I Investigation. PLOS ONE. 2009; 4: e4792. Available from: https://doi.org/10.1371/journal.pone.0004792.
- Fausther-Bovendo H, Kobinger G. Plant-made vaccines and therapeutics. Science 2021; 373: 740–1. Available from: https://doi.org/10.1126/science.abf5375.
- Hellwig S, Drossard J, Twyman RM, Fischer R. Plant cell cultures for the production of recombinant proteins. Nat Biotechnol. 2004; 22: 1415–22. Available from: https://doi.org/10.1038/nbt1027.
- Woo S-D, Roh JY, Choi JY, Jin BR. Propagation of Bombyx mori Nucleopolyhedrovirus in nonpermissive insect cell lines. J Microbiol Seoul Korea. 2007; 45: 133–8.
- Hong M, Li T, Xue W, Zhang S, Cui L, Wang H, et al. Genetic engineering of baculovirus-insect cell system to improve protein production. Front Bioeng Biotechnol. 2022; 10. Available from: https://doi.org/10.3389/fbioe.2022.994743.
- Hu Y-C, Yao K, Wu T-Y. Baculovirus as an expression and/or delivery vehicle for vaccine antigens. Expert Rev Vaccines. 2008; 7: 363– 71. Available from: https://doi.org/10.1586/14760584.7.3.363.
- Fernandes B, Castro R, Bhoelan F, Bemelman D, Gomes RA, Costa J, et al. Insect Cells for High-Yield Production of SARSCoV-2 Spike Protein: Building a Virosome-Based COVID-19 Vaccine Candidate. Pharmaceutics. 2022; 14: 854. Available from: https://doi.org/10.3390/pharmaceutics14040854.
- Struble LR, Smith AL, Lutz WE, Grubbs G, Sagar S, Bayles KW, et al. Insect cell expression and purification of recombinant SARSCOV-2 spike proteins that demonstrate ACE2 binding. Protein Sci. 2022; 31: e4300. Available from: https://doi.org/10.1002/pro.4300.
- Li T, Zheng Q, Yu H, Wu D, Xue W, Xiong H, et al. SARS-CoV-2 spike produced in insect cells elicits high neutralization titres in non-human primates. Emerg Microbes Infect. 2020; 9: 2076–90. Available from: https://doi.org/10.1080/22221751.2020.1821583.
- Fernandes B, Sousa M, Castro R, Schäfer A, Hauser J, Schulze K, et al. Scalable Process for High-Yield Production of PfCyRPA Using Insect Cells for Inclusion in a Malaria Virosome-Based Vaccine Candidate. Front Bioeng Biotechnol. 2022; 10. Available from: https://doi.org/10.3389/fbioe.2022.879078.
- Mabashi-Asazuma H, Kuo C-W, Khoo K-H, Jarvis DL. Modifying an Insect Cell N-Glycan Processing Pathway Using CRISPR-Cas Technology. ACS Chem Biol. 2015; 10: 2199–208. Available from: https://doi.org/10.1021/acschembio.5b00340.
- Strange K, Christensen M, Morrison R. Primary culture of Caenorhabditis elegans developing embryo cells for electrophysiological, cell biological and molecular studies. Nat Protoc. 2007; 2: 1003–12. Available from: https://doi.org/10.1038/nprot.2007.143.
- Espejo L, Hull B, Chang LM, DeNicola D, Freitas S, Silbar V, et al. Long-Term Culture of Individual Caenorhabditis elegans on Solid Media for Longitudinal Fluorescence Monitoring and Aversive Interventions. JoVE J Vis Exp. 2022: e64682. Available from: https://doi.org/10.3791/64682
- Hobbs DJ, Fryer SE, Duimstra JR, Hedstrom OR, Brodie AE, Collodi PA, et al. Culture of Cells from Juvenile Worms of Schistosoma mansoni. J Parasitol. 1993; 79: 913–21. Available from: https://doi.org/10.2307/3283730.
- Lazcano-Perez F, Roman-Gonzalez SA, Sanchez-Puig N, Espinosa RA-. Bioactive Peptides from Marine Organisms: A Short Overview. Protein Pept Lett. 2002; 19: 700–7. Available from: https://doi.org/10.2174/092986612800793208.
- Jayakrishnan A, Wan Rosli WR, Tahir ARM, Razak FSA, Kee PE, Ng HS, et al. Evolving Paradigms of Recombinant Protein Production in Pharmaceutical Industry: A Rigorous Review Sci. 2024; 6: 9. Available from: https://doi.org/10.3390/sci6010009.
- Cid R, Bolívar J. Platforms for Production of Protein-Based Vaccines: From Classical to Next-Generation Strategies. Biomolecules. 2021; 11: 1072. Available from: https://doi.org/10.3390/biom11081072.