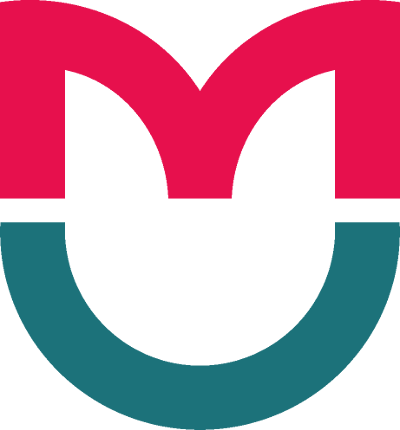
This article is an open access article distributed under the terms and conditions of the Creative Commons Attribution license (CC BY).
ORIGINAL RESEARCH
Production and biological activity of the exogenous mRNA encoding human MxA protein
1 Smorodintsev Research Institute of Influenza of the Ministry of Health of the Russian Federation, St. Petersburg, Russia
2 Institute of Cytology, Russian Academy of Science, St. Petersburg, Russia
Correspondence should be addressed: Sergey A. Klotchenko
Professora Popova, 15/17, St. Petersburg, 197022, Russia; ur.liam@kitafsof
Funding: the study was supported by the Russian Science Foundation, Agreement No. 23-25-00433, project title: “Assessment of antiviral effect of the mRNA encoding human MxA protein” (manager M.A. Plotnikova), https://rscf.ru/project/23-25-00433/
Author contribution: Plotnikova MA — study design, experimental procedure, analysis of the results, statistical processing, manuscript writing; Bobkov DE — confocal microscopy examination; Klotchenko SA — production and characterization of the exogenous mRNA preparations, manuscript editing.
The Mx proteins induced by type I and III interferons are the important innate immunity mediators and are involved in protection against various RNA and DNA viruses [1]. These proteins belonging to the large hydrolase enzyme family (GTPases) are homologous to vertebrates [2, 3].
In humans, two different Mx-GTPases referred to as MxA and MxB are encoded on chromosome 21. Both proteins are localized to the cytoplasm and show a characteristic granular staining pattern when detected by the immunofluorescence method [3]. Human MxA is a cytoplasmic protein with the weight of 78 kDa that is closely linked with the smooth endoplasmic reticulum [1]. MxA has a comparatively wide antiviral spectrum against various types of viruses, regardless of their intracellular replication type. The MxA-sensitive viruses include representatives of the bunyaviruses, orthomyxoviruses, paramyxoviruses, rhabdoviruses, togaviruses, picornaviruses, and hepatitis B virus, a DNA virus with the genomic RNA intermediate [2, 4, 5]. It is interesting that some viruses are inhibited in the cell-type specific manner. This suggests that unknown cellular factors may affect antiviral specificity [6].
In addition to antiviral activity, recent studies defined the role of MxA in various cancer types, specifically breast cancer and prostate carcinoma [1]. Thus, it has been reported that high MxA levels and large amounts of tumor-infiltrating lymphocytes are independent prognostic factors of relapse-free survival in patients with triple-negative breast cancer [7]. It has been also reported that MxA expression by tumor cells more often results in metastasis-free survival after adjuvant chemotherapy [8]. In this regard, it is expected that artificial increase in tumor MxA levels can lead to favorable outcomes in cancer patients and make chemotherapy more effective. All the above suggests that MxA is an extremely promising therapeutic agent.
Since MxA is an intracellular protein, and delivery of proteins of such size into cells remains a major problem, we have proposed a relatively new approach involving the use of the human MxA protein-encoding exogenous mRNA for investigation of the MxA biological properties. This concept has been actively developing in the past decade; it was quite successfully implemented in 2020 when creating the next generation of mRNA vaccines against COVID-19 produced by Pfizer/BioNTech and Moderna. Considerable progress was made in regenerative medicine, where mRNA is used to reprogram somatic cells, as well as in protein replacement therapy used for treatment of genetic disorders.
The study aimed to construct and produce the exogenous mRNA encoding the functional human cytoplasmic MxA protein by IVT; to study its translational properties; to assess and identify the patterns of the expression of some interferon system genes (IFN-stimulated genes) in response to introduction of the exogenous mRNA into cells. In the future we suppose to assess the therapeutic potential of the mRNA construct developed against influenza A and B viruses, respiratory syncytial virus, and coronavirus SARS-CoV-2.
METHODS
Construction of plasmid vectors
The nucleotide insertions encoding human MxA protein were obtained from the total RNA preparations extracted from the A549 cells by RT-PCR using the primers (GAGAGCGGCCGCGCCACCATGGTTGTTT/ ATCTTCTAGATTAACCGGGGAACTGGGCAAGC) comprising restriction sites for the Not I and Xba I endonucleases. The fragments of appropriate size (2017 bp) were inserted in the pJET1.2 vector system comprising the T7 promoter region by the Not I/Xba I restriction with subsequent sticky-end ligation. To produce the construct with the cap-independent translation, the IRES-element sized 605 bp was amplified in a similar way using the pIRESneo3 plasmid vector as a template; the poly(A) sequences, 5′ and 3′ UTR sized 114, 57, and 110 bp, respectively, were synthesized by Evrogen (Russia). All untranslated cassette components were ligated into the pJET1.2 vector in accordance with the design proposed (see Results).
The colonies obtained by cloning were screened by RT-PCR. The plasmid constructs containing insertions of the expected size were accumulated and purified using the Plasmid Miniprep 2.0 kit (Evrogen; Russia). The sequences of the plasmid constructs developed were confirmed using Sanger sequencing by Evrogen (Russia).
In vitro transcription
Preparations of the exogenous mRNAs (mRNA-MxA-CDS, mRNA-MxA-IRES, mRNA-MxA-UTR, and mRNA-GFP-CDS) were obtained by IVT using the HighYield T7 ARCA mRNA Synthesis Kit (#RNT-102, Jena Bioscience; Germany). A total of 1 µg of the plasmid linearized by the Xba I site was used as a template for RNA synthesis. The synthetic Anti-Reverse Cap Analog (ARCA) added to the reaction mixture to the ratio of ARCA/GTP 4 : 1 was used as a cap. The modified nitrogenous bases, 5-methylcytidine (#NU-1138, 5-Methyl-CTP, m5C, Jena Bioscience; Germany) and pseudouridine (#NU-1139, Pseudo-UTP, Ψ, Jena Bioscience; Germany), were used in the reaction. The reaction was carried out in accordance with the manufacturer’s protocol.
After the RNA synthesis, the DNA template was removed by further cleavage with the Turbo DNAse (#AM1345, Thermo Fisher Scientific; USA). The Poly(A) Tailing Enzyme Testkit (#RNT-004, Jena Bioscience; Germany) was used for the nontemplated 3’ polyadenylation of the transcribed mRNAs. The resulting transcripts were purified using the columns, the RNA Clean & Concentrator kit (#R1017, Zymo Research; USA). The resulting mRNA concentrations were measured with the NanoDrop-1000 spectrophotometer and Qubit 4 fluorometer (Thermo Fisher Scientific; USA).
Agarose gel electrophoresis
The plasmid DNA preparations were analyzed in the 0.8% agarose gel prepared based on the 1× TAE buffer containing up to 0.5 µg/mL of ethidium bromide using the 6× buffer for DNA application.
The mRNA samples were analyzed under denaturing conditions in the 1% agarose gel containing 0.5 µg/mL of ethidium bromide. A total of 500 ng of mRNA sample were mixed with the equal amount of the Gel Loading Buffer II (#AM1344, Invitrogen; USA), heated for 5 min at 80 °C, and applied to the gel.
The electrophoretic separation results were imaged using the Gel Doc EZ Imager (Bio-Rad; USA).
Cell culture maintenance and transfection with the exogenous mRNAs
The following cultures were used in the study for various experiments: the passaged culture of the A549 cells (human lung carcinoma) obtained from the ATCC collection (USA) and the culture of the MDCK FR58 cells (canine kidney cells) obtained from the IRR collection (USA).
The A549 cells were cultured in the F12K growth medium (Gibco; USA) in the presence of the 10% КРС serum (Gibco; USA); the MDCK cells were cultured in the alpha-MEM medium (BioloT; Russia) added КРС serum to 5%. The culture maintenance and all the experiments were implemented without adding antibiotics.
To ensure transfection with the exogenous mRNAs, we used the 24 h 90–100% cell monolayer; the growth medium was replaced with the serum-free medium immediately before adding mRNA. Cells were transfected using the commercially available Lipofectamine MessengerMAX transfection reagent (Thermo Fisher Scientific; USA) in accordance with the manufacturer’s instructions. The mRNA complexes were added to the wells of the 96-well plate in a volume of 10 µL, and to the wells of the 12-well plate in a volume of 50 µL. There were 100 ng of mRNA and 0.3 µL of the Lipofectamine MessengerMAX reagent per well of the 96-well plate, 450 ng of RNA and 1 µL of the Lipofectamine MessengerMAX reagent per well of the 12-well plate. Depending on the objectives of the experiment, cells were incubated with the RNA/Lipofectamine complexes for 4–24 h at 37 °C and 5% СО2.
Confocal miscroscopy
Confocal imaging of the fixed cells was performed 24 h after transfection with the exogenous mRNAs. For that the cell monolayer grown on the glass slides was washed with DPBS, fixed with the 4% paraformaldehyde solution for 10 min, and permeabilized with the 0.1% Triton X-100. Blockage was accomplished using the 1% BSA solution in DPBS. The nuclei were stained with DAPI (AppliChem; USA), and the actin cytoskeleton was stained with phalloidin covalently linked to rhodamine (Thermo Fisher Scientific; USA). The human MxA protein imaging was performed using the primary MxA/Mx1 Antibody labeled with biotin (Novus Biologicals; Germany) with subsequent development using Streptavidin DyLight 633 (Thermo Fisher Scientific; USA). Cell microscopy was performed using the TCS SP8 inverted confocal laser scanning microscope (Leica; Germany).
Enzyme-linked immunosorbent assay (ELISA)
The MxA protein levels were measured using the Human MxA Protein ELISA kit (BioVendor; Czech Republic) in accordance with the manufacturer’s instructions. The results were recorded in the dual-wavelength mode (at the primary wavelength of 450 nm and reference wavelength of 655 nm) using the CLARIOstar microplate reader (BMG Labtech; Germany)
Expression level assessment
The IFN-stimulated gene expression levels were assessed by real-time RT-PCR using the previously designed primers [9]. The total RNA preparations were extracted using the TRIzol reagent (Thermo Fisher Scientific; USA) and then treated with DNAse (Biolabmix; Russia). The RT reaction was carried out using the RNAscribe RT kit (Biolabmix; Russia), dT(16) primers and 2 µg of the DNA-free RNA. PCR was conducted using the ready-made BioMaster HS-qPCR (2×) kit (Biolabmix; Russia), to which 1–2 µL of cDNA were added.
Relative gene expression was calculated by the double delta Ct (ΔΔCt) method using GAPDH and ACTB as the normalizing genes. The relative gene expression levels were determined using the inductive formula R = 2–[ΔΔCt]. Calculations were performed using the Microsoft Office Excel 2003/2007 software (USA).
Statistical data processing
Significance of differences was assessed with the GraphPad Prism 6 software tool (GraphPad Software; USA) using the nonparametric Kruskal–Wallis test (to assess significance of differences between three or more independent groups) and the Dunnett's test (for multiple intergroup comparisons). The differences were considered significant at р < 0.05.
RESULTS
Functional mRNA production
According to the NCBI database [10], four MxA proteinencoding mRNA transcript variants were identified in humans.
Despite the differences in length and 5′ UTR variation, the transcript variants 1, 2 and 3 encode the same MxA protein isoform referred to as isoform a. The transcript variant 4 has an alternative 5′ UTR and does not comprise three exons in the 3′ coding region, which leads to the frameshift, due to which this mRNA encodes the shorter MxA protein isoform (b), also known as varMxA [11]. When designing the constructs encoding mRNA of human MxA gene, primers for specific cloning of the canonical MxA protein form (a) were selected.
The human MxA protein-encoding sequence was obtained from the total RNA preparation (after DNAse treatment) by RT-PCR (Pfu DNA polymerase) using the selected primers. According to the sequencing results (fig. 1А), the sequence was completely identical to the expected canonical MxA protein form (a), but it had a single reported amino acid variation V379I having no effect on the MxA protein physical and chemical properties [12].
To generate mRNA by the IVT method, three plasmid constructs were proposed (fig. 1B) comprising the promoter region specific for the phage T7 polymerase as a mandatory component of the cassette. One of the constructs comprised the MxA protein-encoding sequence (mRNA-MxA-CDS) only, while untranslated regions potentially enhancing the effectiveness of translation of the protein encoded by these mRNAs were additionally introduced to the other two. To ensure realization of the cap-independent translation, the second mRNA-MxA-IRES was constructed complemented with the cropped type II IRES element from the encephalomyocarditis virus (EMCV) comprising an A7 bifurcation sequence on the 5′ end [13, 14]. The third mRNA-MxA-UTR comprised additional untranslated regions on the 5′ and 3′ ends, as well as the region ensuring the templated extension of the poly(A) tail during IVT.
All three proposed plasmid constructs were successfully generated and accumulated; the expression cassette sequencing showed that these were completely matched to the expected sequences. Then the plasmids were linearized (the electrophoretic separation results are provided in fig. 1C) and used as a DNA template for IVT.
The mRNAs we had generated (fig. 2А) comprised the synthetic cap analog (ARCA), the modified bases pseudouridine (Ψ) and 5-methylcytidine (m5C), which, according to modern literature data, reduce the exogenous transcript immunogenicity [15, 16], as well as the 3’ poly(A) tail. The latter was added in the templated manner (when the cassette was used for mRNA-MxAUTR) or by conducting a separate polyadenylation reaction (for mRNA-CDS and mRNA-IRES). In addition, we also produced the exogenous mRNA encoding the green fluorescent protein (GFP): mRNA-GFP-CDS containing no untranslated regions, the structure of which was equivalent to that of mRNA-MxA-CDS. The amounts of the resulting MxA protein-encoding mRNAs reached 200 µg, and the concentrations were up to 350 ng/µL.
Assessment of the exogenous mRNA-encoded protein product translation
Translational activity of the exogenous mRNAs produced was assessed by transfection of the MDCK cells. The use of ELISA and the results obtained in a series of experiments showed that mRNA-МхА-IRES was incapable of translation in the studied cell line: when using this mRNA, we failed to detect the MxA protein throughout 36 h after the mRNA introduction into cells. However, the diagnostically significant amounts of human MxA protein were detected in the cell lysates as early as 4 h after transfection of the MDCK cells with two other mRNAs (results not shown).
When using both mRNA-MxA-CDS and mRNA-MxA-UTR, the human MxA protein concentrations were comparable and reached 20 ng/mL 20 h after transfection (fig. 2B).
Internalization and intracellular localization of human MxA protein encoded by the exogenous mRNAs were studied by confocal microscopy. As shown in fig. 3, the MxA protein was effectively translated by the MDCK cells 24 h after the mRNA introduction. According to the ELISA results, MxA encoded by both exogenous mRNAs was found exclusively in the cell cytoplasm, it was spread diffusely in the form of the characteristic granules.
Assessment of the IFN-stimulated gene expression in response to introduction of the exogenous mRNA-MxA-UTR
It is well known that introduction of synthetic exogenous mRNAs can lead to activation of the cytoplasmic RNA sensors and activate the immune response. The MxA protein also can modulate production of the IFN-stimulated genes, as one of the most important effector metabolites of the innate immunity. We assessed specificity of such response 4 h and 24 h after transfection of the A549 cells with mRNA-MxA-UTR using mRNA-GFP-CDS as a non-specific stimulation control (fig. 4). Thus, 4 h and 24 h after transfection of cells with mRNA-MxA-UTR the detected relative MxA mRNA level was about 6500 times higher compared to the level in the control cells (taken as the unit) (fig. 4А). It has been also shown that the exogenous mRNA introduction into cells results in the non-specific (not dependent on the exogenous mRNA type) upregulation of OAS1, as well as the cytoplasmic sensor genes MDA5 and RIG-I, which suggests the innate immune response activation. It is interesting that transfection of cells with mRNA-MxA-UTR led to almost 1000-fold decrease in the PKR levels compared to intact cells, while the trend towards a 4-fold increase in activity of this gene was reported for mRNA-GFP-CDS.
DISCUSSION
The protein-encoding exogenous mRNAs represent a promising tool that makes it possible to conduct fundamental research on the patterns of functioning, signaling, and metabolism of proteins in cells and has a huge therapeutic potential. The human MxA protein-encoding mRNA preparations we have constructed and synthesized will be used to assess their antiviral activity against respiratory viruses in the future.
Synthetic mRNAs have the same structure as natural mRNA molecules: 5’ cap (more often its structural analog), 5′ and 3′ UTRs flanking the protein-coding region, and poly(A) tail [17, 18].
Of the three proposed mRNAs, we managed to reliably demonstrate stable translation of two constructs, one of which comprised 5′ and 3′ UTRs, and the other one comprised no service regions in addition to the protein-coding part (except for the cap analog and poly(A) tail). Surprisingly, despite the increased effectiveness of the IRES-containing mRNA translation reported in the literature [19], there was no protein synthesis with the mRNA-MxA-IRES we had proposed. In our study, we used the IRES element of EMCV belonging to the Picornaviridae family not using cap during replication. The resulting mRNA construct comprised both cap analog and the IRES element. Presumably, the simultaneous presence pf these two ribosome recognition sites in the close proximity results in blockage of the cap-dependent translation from this transcript.
In the future we nevertheless plan to assess translation of synthetic mRNA-MxA-IRES comprising no cap analog. According to the literature data, despite the fact that the IRES-dependent translation is less effective compared to the cap-dependent translation under normal conditions, the IRESmediated translation can persist and outperform the translation involving cap under conditions of cellular stress (including heat shock, viral infection, etc.) [20].
It is well known that the 5′ and 3′ UTRs are among key regulators of the mRNA molecule intracellular kinetics. In particular, mRNAs having long 3′ UTRs have a shorter half-life, while mRNAs having short 3′ UTRs are less effectively translated [18]. Comparison of MxA protein levels in the MDCK cells 24 h after transfection with the exogenous mRNAs revealed no advantage in the translation activity resulting from the presence or absence of UTRs in the mRNAs. Both mRNAs capable of translation led to protein production (within 20 ng/mL) 24 h after transfection. Immunofluorescence analysis of the MDCK cells transfected with the exogenous mRNAs showed that these contained the granules, presumably having a ring configuration (visible at maximum magnification), which were diffusely distributed in the cytoplasm. This finding is entirely consistent with the published data on the fact that the MxA homooligomerization occurs at physiological salt concentrations in the cell in vivo, and such aggregation prevents degradation and ensures stability of protein with the half-life exceeding 24 h [21]. Our findings suggest that the MxA protein produced will function in the cells like native.
When assessing antiviral potential of the MxA protein translated from the exogenous mRNA, special attention should be paid to the non-specific innate immune response activation resulting from the increased immunogenicity of the mRNA molecule. It is well known that the pattern recognition receptors, such as transmembrane TLR3, 7, 8, 9, and the RIG-I and MDA5 cytoplasmic sensors can recognize foreign nucleic acids and lead to the reciprocal expression of pro-inflammatory cytokines or activation of inflammation [22]. We have shown upregulation of the RIG-I and MDA5 cytoplasmic sensors, as well as the OAS1 IFNstimulated gene 24 h after transfection of cells with the exogenous mRNAs. The expression patterns of these genes differed in strength and correlated with each other for various exogenous mRNAs. We assume that such alterations result from non-specific immunogenicity of the exogenous mRNA. The GFP-encoding mRNA had the greatest effect on the expression of the studied genes, which can be due to its structure or due to the fact that negative regulation of the expression of these genes associated with the negative feedback loop is possible in case of mRNA-MxA.
It is interesting that transfection of the A549 cells with mRNA-MxA-UTR caused the prolonged (at least up to 24 h) potent specific suppression of PKR at the transcription level as early as after 4 h.
CONCLUSIONS
During the reported study: 1) the MxA protein-encoding mRNAs capable of effective translation in the eukaryotic systems were produced using the plasmid constructs we had designed as a template in the IVT reactions; 2) up to 20 ng/mL of the MxA protein product were accumulated within 24 h after transfection of cells with the exogenous mRNAs; 3) the diffuse intracellular MxA protein distribution in the cells was confirmed; 4) the expression of cytoplasmic sensors and some IFN-stimulated genes in response to introduction of the human MxA protein-encoding exogenous mRNA in the cells was assessed. The authors’ further research will be focused on assessing the therapeutic potential of the designed mRNA constructs against influenza A and B viruses, respiratory syncytial virus, and coronavirus SARSCoV-2. Assessment will primarily involve the cell-based models of infections caused by the above pathogens; if the therapeutic potential is high, we plan to assess antiviral activity of the MxA protein-encoding exogenous mRNAs in the murine model of influenza pneumonia.