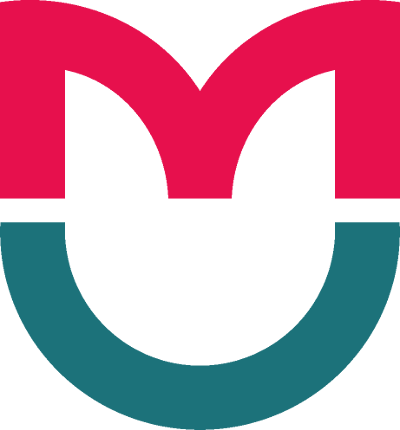
ORIGINAL RESEARCH
Genetic polymorphism of Staphylococcus epidermidis strains in patients of the Neonatal Intensive Care Unit
1 Department of Microbiology and Clinical Pharmacology,
Kulakov Research Center for Obstetrics, Gynecology and Perinatology, Moscow, Russia
2 Department of Clinical and Molecular Genetics,
Kulakov Research Center for Obstetrics, Gynecology and Perinatology, Moscow, Russia
3 Department of Neonatology and Pediatrics,
Kulakov Research Center for Obstetrics, Gynecology and Perinatology, Moscow, Russia
Correspondence should be addressed: Alexey B. Gordeev
ul. Akademika Oparina, d. 4, Moscow, Russia, 117997; ur.sertorp.agev@weedrog
Funding: this study was supported by the Grant Agreement No. 14.607.21.0019 of the Ministry of Science and Education of the Russian Federation
dated June 5, 2014 (ID 2014-14-579-0001-065).
Contribution of the authors to this work: Gordeev AB — bioinformatics analysis of nucleotide sequences, analysis of literature, drafting of a manuscript; Lyubasovskaya LA — micribiological studies, analysis of literature, data interpretation, drafting of a manuscript; Rodchenko JV, Dubodelov DV — microbiological studies, drafting of a manuscript; Mukosey IS, Kochetkova TO — whole genome suquencing and genome assembling; Nikitina IV — research planning, data interpretation; Ionov OB, Zubkov VV — research planning, organization of biomaterial sampling; Trofimov DYu — research planning, organization of whole genome sequencing; Priputnevich TV — research planning, organization of microbiological studies, data interpretation, drafting of a manuscript. All authors participated in editing of the manuscript.
Staphylococcus epidermidis is a member of the normal flora inhabiting human skin and mucosa [1, 2]. Still it can cause life-threatening diseases, such as pneumonia, sepsis and catheter-related infections, in neonates with low and extremely low body weight. The pathogenic potential of these bacteria varies over a very wide range, from virulence to commensalism. In spite of advances in our understanding of pathogenic mechanisms used by S. epidermidis, they still remain understudied.
S. epidermidis strains have different genetic properties. A method of multilocus sequence typing (MLST) has been developed and is actively used to classify bacterial strains into sequence types. Although the number of S. epidermidis sequence types is quite big, the majority of the nosocomial strains from around the world can be grouped to form a single clonal complex (CC2) that comprises closely related sequence types [2, 3]. Many of S. epidermidis strains circulating in the hospital environment are resistant to methicillin; this type of antibiotic resistance is associated with mobile genetic elements referred to as staphylococcal cassette chromosome mecs (SCCmec) [1, 4]. Resistance to methicillin is mediated by the mecA gene that encodes an alternative penicillin-binding protein with reduced affinity to methicillin (oxacillin). Methicillin resistance is a pressing clinical issue due to the fact that mecA of staphylococcal strains confers resistance to all beta-lactam antibiotics (penicillins, including protected penicillins, cephalosporins and carbapenems). Gene sets contained in SCCmec elements may vary and include genes encoding resistance to other antibiotics, as well as insertion sequences, such as IS431, plasmids, such as pT181, and transposons, e. g. Tn554.
Nosocomial strains of S. epidermidis also tend to be resistant to aminoglycosides and macrolides and, to a lesser degree, to tetracycline, chloramphenicol, vancomycin and clindamycin [5]. According to some researchers, methicillin (oxacillin) resistance is observed in over 70 % of S. epidermidis nosocomial strains [6, 7]. Antibiotic resistance genes are often found in mobile genetic elements of S. epidermidis. On the whole, bacterial strains demonstrate a strong correlation between the presence of resistance genes and resistance phenotypes [1, 8].
S. epidermidis strains harbor a few virulence determinants in their genome, i. e. genes encoding synthesis of proteins that promote infection and sustain bacterial viability. Although some works report the association between bacterial virulence and virulence factors, it is currently impossible to identify markers that could be used to accurately differentiate between pathogenic and harmless strains [1]. Perhaps, advances in this field depend on the study of complex interactions between bacteria and the immune system of humans. Some virulence factors are part of the core genome and can be found in all strains of S. epidermidis. Other factors associated with pathogenicity are harbored by few strains. One of the approaches used to identify genetic features of microbial strains relies on high-throughput whole-genome sequencing that allows performing a comprehensive analysis of genetic properties of selected strains and also detecting a large number of genes or their variants by conducting a bioinformatic analysis of the obtained assemblies. Here, in Kulakov Research Center for Obstetrics, Gynecology and Perinatology we study genetic diversity of various microbial strains that circulate in the hospital environment [2, 9].
The aim of this work was to study genetic diversity of nosocomial strains of S. epidermidis isolated from patients of the Neonatal Intensive Care Unit of Kulakov Research Center for Obstetrics, Gynecology and Perinatology.
METHODS
We isolated 14 strains of S. epidermidis from patients of the Neonatal Intensive Care Unit of Kulakov Research Center for Obstetrics, Gynecology and Perinatology. Strains 3, 7, 8, 13, and 14 were isolated from tracheobronchial aspirates of 5 neonates; strains 1, 5, and 9 were isolated from stool samples of 3 neonates; strains 4 and 10 were isolated from oropharyngeal swabs of 2 patients; strains 2 and 11 were isolated from blood samples of 2 neonates; strain 12 was isolated from eye discharge of 1 patient; strain 6 was isolated from the lung tissue of one neonate postmortem. All neonates had signs of infection (pneumonia, sepsis, conjunctivitis) at the time of sample collection; autopsy of the dead neonate confirmed pneumonia. All neonates had low or extremely low body weight.
Specimens were inoculated into Columbia agar base (Bio-Rad, USA) supplemented with 5 % sheep blood (Eco-Lab, Russia) and mannitol salt agar (Liofilchem, Italy). Plates were incubated in the thermostat at 36–37 °C for 48 hours.
Isolates were identified to a species level using matrix-assisted laser desorbtion/ionization time-of-flight mass spectrometry (MALDI-TOF MS). Sample preparation is described below. One-day old microbial cultures (1–2 colonies) were applied onto a stainless steel target plate, allowed to dry for 1–2 minutes, and then covered with 2 μL saturated matrix solution (a saturated solution of alpha-cyano-4-hydroxycinnamic acid by Bruker Daltonics, Germany, in 50 % acetonitrile and 2.5 % trifluoroacetic acid). All reagents including water were either analytical or mass spectrometry grade. Crystals were exposed to air for 5–10 min to dry. Humidity and temperature were not controlled. Two replicates were run for each sample. Mass spectrometry was performed using MALDI-TOF mass analyzer Autoflex III (Bruker Daltonics, Germany) equipped with a nitrogen laser of 337 nm wavelength. All measurements were performed in linear mode with positive ion detection. To accumulate mass spectra, laser power was set to a minimum threshold sufficient for sample desorbtion/ionization. Mass analyzer settings were optimized for the m/z range of 2,000 to 20,000. External calibration was performed using reference masses of known Escherichia coli proteins. The sample was applied onto 3 steel targets; the spectrum was recorded as a sum of 10 measurement runs, with 50 laser bursts per run. To record, process and analyze the spectra, flexControl 2.4 (Build 38) and flexAnalysis 2.4 (Build 11) software by Bruker Daltonics, Germany, was used. Mass accuracy was ± 2 Dа. Cluster analysis and comparison of the obtained mass spectra with the values retrieved from mass spectrometry reference databases were performed using Biotyper 1.1 software (Bruker Daltonics, Germany).
Antibiotic sensitivity tests were performed on the automated microbial identification system Vitek 2 Сompact (bioMérieux, France) according to the manufacturer’s protocol. The samples were tested for their sensitivity to benzylpenicillin, cephoxitin, gentamycin, clindamycin, erythromycin, vancomycin and fusidic acid. Results were interpreted using the software supplied by the manufacturer based on the interpretation criteria recommended by EUCAST in 2015.
Genomic DNA was extracted from freshly grown cultures using lysozymes and protein kinase K. DNA was further purified by phenol-chloroform extraction. To obtain DNA libraries, we used Ion Xpress Plus Fragment Library Kit and Ion Xpress Barcode Adapters (Thermo Fisher Scientific, USA) following the manufacturer’s protocol. Quality of the libraries was tested on Agilent 2100 Bioanalyzer system using HighSense DNA Kit (Agilent Technologies, USA) according to the manufacturer’s protocol. Emulsion polymerase chain reaction (PCR) and enrichment of ion sphere particles were performed using Ion OneTouch Template Kit (Thermo Fisher Scientific) according to the manufacturer’s protocol. Sequencing was performed on the Ion PGM Torrent platform using Ion Sequencing Kit and 316 v1 chips (Thermo Fisher Scientific) according to the manufacturer’s protocol. Short reads were assembled into longer sequences (contigs) using MIRA 3 software with the following parameters: job = genome, de novo, accurate.
Sequence typing was performed using MLST 1.8 software [10]. Polymorphism analysis of 7 loci (arcC, aroE, gtr, mutS, pyr, tpi and yqiL) of the assembled contigs was conducted. Antibiotic resistance genes were identified using ResFinder 2.1 software [11]. The minimally acceptable degree of similarity was 90 %, the minimal overlap length was 70 %. Virulence-associated genes and loci were identified by comparing their sequences to the corresponding nucleotide sequences obtained from the GenBank database [12] using BLAST software [13].
RESULTS
We isolated 14 strains of S. epidermidis from the patients of the Neonatal Intensive Care Unit of Kulakov Research Center for Obstetrics, Gynecology and Perinatology. Whole-genome sequence was run for each strain followed by sequence typing, identification and analysis of genes associated with virulence and antibiotic resistance. Results of genome assembly are shown in tab. 1.
Sequence typing identified 8 sequence types among the strains. The most frequent types were ST2 and ST59 (each detected in 4 strains). The rest 6 strains belonged to types ST19, ST22, ST87, ST173, ST210, and ST218. All strains belonged to known sequence types.
A summary of antibiotic resistance genes identified in S. epidermidis is presented in tab. 2 and tab. 3.
S. epidermidis strains were found to harbor amynoglycoside resistance genes, namely aacA-aphD, aadD and aphA. The most frequent of them was aacA-aphD detected in 12 strains (strains 1–12). Gene aadD was also present in the genomes of 5 strains (strains 1, 2, 4, 9, and 10), gene aphA— in the genomes of 2 strains (strains 3 and 7). These genes are believed to form a phenotype resistant to some antibiotics of the aminoglycoside family. According to the recent studies [8], locus aacA-aphD forms a phenotype resistant to amikacin, gentamycin, kanamycin and tobramycin. Gene aadD mediates resistance to amikacin, kanamycin, neomycin and tobramycin, while gene aphA mediates resistance to kanamycin and neomycin. A gentamycin resistance phenotype was observed in thirteen studied strains carrying resistance genes.
The studied strains were found to carry 2 beta-lactam resistance genes. The blaZ gene that encodes beta-lactamase and is capable of cleaving penicillins was present in the genomes of all studied strains. Phenotypically all strains were resistant to benzylpenicillin. Resistance to methicillin in staphylococci is determined by the mecA gene that encodes an alternative penicillin-binding protein with low affinity to methicillin [1, 14]. Gene mecA responsible for methicillin resistance was found in 13 of 14 strains (strains 1–12 and 14). Phenotypically, all strains demonstrated resistance to methicillin (cephoxitin), i. e., were methicillin-resistant.
Of 2 known genes that mediate resistance to fusidic acid, only one — fusB — was detected in the studied strains (strains 2, 4–6, 9, 19). Gene fusC was absent. Of 14 strains, 10 exhibited phenotypic resistance to fusidic acid.
Some researchers demonstrated that staphylococci can harbor vanA genes mediating resistance to vancomycin [8]. However, the strains studied in our experiments did not carry vancomycin resistance genes (vanA, vanB and vanZ), and none of the strains had a vancomycin-resistant phenotype.
Phenotypic resistance to lincosamides (clindamycin) was demonstrated by 7 strains. We identified 2 genes associated with lincosamide resistance. Gene lnu(A) was present in 4 strains (strains 1, 3, 8, 14) while gene vga(B) was found in strain 5 only. Gene vga(A) that can mediate resistance to lincosamides was absent in the studied strains.
Among the studied strains, there were 10 with phenotypic resistance to macrolides (erythromycin). Genomes of strains 6, 7, 11, and 12 harbored the msr(A) gene, genomes of strains 7, 11, and 12 harbored the mph(C) genes. Those genes mediate bacterial resistance to macrolides, including erythromycin.
Of 3 genes responsible for resistance to both macrolides and lincosamides [8, 11], 2 were carried by the studied strains. Gene erm(C) was present in strains 1, 3, 7, 8, 10, and 14; gene erm(A) was present in one strain (strain 5). Gene erm(B) was absent in the studied genomes.
For an adequate therapy of S. epidermidis-associated infections, phenotypic resistance of its strains to antibiotics must be tested. In our work we attempted to identify genes that mediate resistance to different groups of antibiotics. Of particular interest are genes that determine bacterial resistance to drugs that have not been included in treatment regimens so far. First, there is a chance of horizontal gene transfer of mobile genetic elements and plasmids between S. epidermidis and other bacteria. Second, the presence or absence of resistance genes in the genome of the studied strain makes it possible to assess its genetic characteristics.
The vat(B) gene that mediates resistance to streptogramin B and possibly lincosamides [8] was observed in only one strain (strain 5). Of 2 genes conferring tetracycline resistance, only one gene tet(K) was detected in the studied genomes (strains 1, 7, 8, and 14). The other gene tet(M) was not observed in the studied strains. Strains 1 and 3 carried gene dfr(G) associated with trimethoprim resistance. Another trimethoprim resistance gene dfr(K) was not present in the genomes.
Results of virulence-associated gene search in S. epidermidis are shown in tab. 4.
DISCUSSION
In spite of sequence type diversity observed in the studied strains (8 variants were detected), 10 strains of 14 belonged to the major clonal complex CC2. These strains belonged to ST2, ST22, ST59 and ST87 sequence types. According to the literature, ST59 and ST22 are the most common sequence types of S. epidermidis circulating in the hospital environment [2, 3]. Sequence type ST2 is an progenitor source for one of 2 major clusters (cluster I) of the CC2 clonal complex. A multicenter study carried out by Miragaiet et al. in 2007 in 18 countries demonstrated worldwide dissemination of S. epidermidis clonal lineages in the hospital environment [3]. Similar results were obtained in Russia in 2013 [2]. This prompts a supposition that types belonging to the CC2 clonal complex have certain genetic characteristics that allow them to circulate and persist in hospitals for a long time, such as increased resistance to antibiotics or a special combination of antibiotic resistance genes in chromosomal elements, increased ability to form biofilms, etc. Further in-depth research is required aimed to analyze genetic properties of CC2 strains isolated from clinical samples.
The studied staphylococcal strains carry a varying range of genes conferring resistance to antimicrobial drugs. As confirmed by other studies [1, 6, 7, 14], different strains have different sets of resistance genes. Penicillin resistance genes were detected in all 14 strains (100 %); methicillin resistance genes were detected in 13 (93 %) strains; aminoglycoside resistance genes — in 12 strains (86 %); macrolide resistance genes – in 10 strains (71 %); lincosamide resistance genes — in 7 strains (50 %); fusidic acid resistance genes — in 6 strains (43 %); tetracycline resistance genes — in 4 strains (29 %); trimethoprim resistance genes — in 2 strains (14 %). None of the strains carried vancomycin resistance genes. Some strains carried a set of 1 (strain 13) to 8 (strains 1 and 7) antibiotic resistance genes.
It should be noted that the presence of a resistance gene does not imply its expression in the amount sufficient to form a phenotype. However, a nucleotide sequence can either directly form a resistance phenotype or serve as a source for further evolution that will ultimately yield phenotypic resistance.
On the other hand, resistance genes are not the only source of phenotypic antibiotic resistance. Resistance may be associated with modifications or inactivation of native enzymes triggered by mutations in the native genes. For example, staphylococcal resistance to methicillin may be associated not only with the presence of cassette chromosome mecs but also with modifications of the penicillin-binding protein. Besides, reduced sensitivity to antibiotics may be a result of increased activity of efflux pumps that pump antibiotics out of the cell. There are other known types of antibiotic resistance mechanisms. In this work, we did not aim to analyze nucleotide sequences or their localization, detect mutations capable of affecting gene expression or evaluate gene expression levels. The presence of resistance genes positively correlated with phenotypic resistance to antibiotics in penicillin, lincosamides, and macrolides. Phenotypic resistance to other antibiotics was demonstrated by those strains that carried resistance genes and those that did not, meaning that bacteria can employ different resistance mechanisms. Therefore, our research is going on.
To sum up, nosocomial strains isolated from patients of the Neonatal Intensive Care Unit of Kulakov Research Center for Obstetrics, Gynecology and Perinatology carry a wide range of antibiotic resistance genes.
The number of virulence-associated genes found in S. epidermidis strains is fairly large. However, the majority of these genes can promote both pathogenicity and commensalism. Therefore, the presence of such genes in S. epidermidis does not allow us to conclude whether the strain is pathogenic or harmless [1, 14, 15]. However, we did observe a correlation between the presence of virulence factors and pathogenicity of nosocomial strains [16, 17, 18].
All 14 strains carried genes aae and atlE. Both of them encode bifunctional proteins that can act as autolysins and adhesins and participate in biofilm formation. These genes were very common for the studied S. epidermidis strains. Other genes associated with virulence were found only in some of the studied strains. The studied strains carried genes aap and embp and genes icaA and icaD of the ica-operon. Gene aap encodes protein Aap, which is the most important factor of protein-dependent biofilm formation. Gene embp participates in biofilm formation, and genes of the ica-operon mediate synthesis of exopolysaccharide intracellular adhesins that have a role in biofilm formation and immune evasion.
Insertion sequence IS256 was found in some strains, positively correlating with strain virulence [17].
Thus, a set of genes associated with virulence varied in different nosocomial strains isolated from patients of the Neonatal Intensive Care Unit of Kulakov Research Center for Obstetrics, Gynecology and Perinatology. Some strains of S. epidermidis harbored from 4 (strains 1, 3, 5, 7, 11, 12, and 13) to 7 (strains 8 and 14) genes associated with virulence. Interestingly, strains 1 and 7 carried the highest number of resistance genes and the lowest number of virulence genes.
CONCLUSIONS
We have studied genetic diversity of 14 nosocomial strains of S. epidermidis isolated from patients of the Neonatal Intensive Care Unit of Kulakov Research Center for Obstetrics, Gynecology and Perinatology. The strains were classified into 8 sequence types; 10 of 14 strains belonged to the major clonal complex CC2. The studied strains were found to carry a wide range of antibiotic resistance genes and virulence genes. We identified 15 different genes conferring resistance to aminoglycosides, beta-lactams, fusidic acid, macrolides, lincosamides, streptogramin B, tetracycline, and trimethoprim. We also identified genes associated with virulence (aae, atlE, aap, embp, icaA, icaD) and found that their frequency in the studied strains varied. Additionally, insertion sequence IS256 was identified.