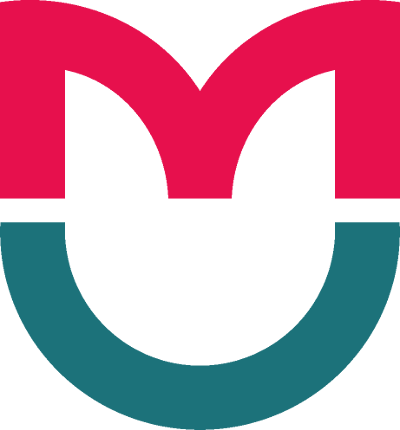
This article is an open access article distributed under the terms and conditions of the Creative Commons Attribution license (CC BY).
ORIGINAL RESEARCH
Taxonomic dysbiosis of gut microbiota and serum biomarkers reflect severity of central nervous system injury
Federal Research and Clinical Center of Intensive Care Medicine and Rehabilitology, Moscow, Russia
Correspondence should be addressed: Ekaterina A. Chernevskaya
Petrovka, 25, s. 2, Moscow, 127051; ur.rrcknf@ayaksvenrehce
Compliance with ethical standards: the study was approved by the Ethics Committee of the Federal Research and Clinical Center of Intensive Care Medicine and Rehabilitology (Protocol № 2/19/2 dated June 20, 2019). Informed consent was obtained from all study participants or their legal representatives.
Author contribution: Chernevskaya EA, Meglei AYu — taxonomic microbiota profiling, serum biomarker tests, data analysis, manuscript preparation; Beloborodova NV — study design, final version of the manuscript; Buyakova IV, Kovaleva NYu, Gorshkov KM — patient recruitment and treatment, clinical data collection; ZakharchenkoVE — sample collection, final version of the manuscript. All authors read and approved the final version of the manuscript.
Over the past decades, advances in intensive care have substantially improved survival in acutely critically ill patients but, at the same time, expanded the population of patients with prolonged dependence on intensive care. This engendered the concept of chronic critical illness (CCI) [1]. CCI criteria include at least 2 weeks in an intensive care unit (ICU); protracted (14–28 days) mechanical ventilation (MV); persistent inflammation; immunosuppression; hypermetabolism and hypercatabolism; increased susceptibility to infection [2–4].
Most survivors of severe brain injury meet CCI criteria. They are also at risk for CCI-attributed neurological deficit aggravated by their underlying condition and caused by functional or metabolic impairment mediated by pro- or noninflammatory factors, including microglia hyperactivation, blood brain barrier (BBB) disruption and altered neurotransmission [3]. These disturbances are detected based on the elevated markers of brain inflammation/injury [5]. Currently, there is no all-in-one biomarker for evaluating the complex pathophysiology of critical illness. Therefore, CCI patient monitoring relies on the whole array of different biomarkers. Of them, the most commonly used are S100 proteins and neuron-specific enolase (NSE) [6–8], which indicate neuronal injury, and non-specific markers of inflammation, like interleukin-6 (IL6) [9] and С-reactive protein (CRP) [10].
Changes in the gut microbiota might be an important contributor to CCI. Microbiota has the ability to affect CNS function in humans, which makes it an attractive object for research [11, 12]. The gastrointestinal (GI) tract may be involved in the development and progression of organ failures in critically ill patients [13, 14]. CCI patients have metabolic disorders leading to protein-energy malnutrition [15] and GI comorbidities caused, among other things, by nasogastric nutrition [16], which could be the underlying cause of dysbiosis observed in such patients [17]. Ever more studies researching the microbiota of critically ill patients are published every year; still, a lot is yet to be elucidated [18].
Human microbiota can be studied using a broad arsenal of methods, from classic culture-based analysis to state-of-the-art next generation sequencing (NGS). Real-time PCR is a promising alternative to culture-based methods that allows creating both qualitive and quantitative profiles of the gut microbiota [19].
The aim of this study was to investigate possible correlations between changes in the taxonomic abundance of the gut microbiota, levels of proinflammatory and neurological serum biomarkers and the severity of CNS injury in patients with CCI.
METHODS
Our prospective observational study included patients with CNS injury of various etiology (n = 29) receiving medical care at the ICU of the Federal Research and Clinical Center of Intensive Care Medicine and Rehabilitology from June 2019 to March 2020.
The following inclusion criteria were applied: patients with CNS injury of various etiology meeting CCI criteria (at least 2 weeks in ICU and/or 14–28 days of MV) aged 18–75 years and admitted to ICU for intensive neurological care. Exclusion criteria: age over 75 years; chemotherapy/hormonal therapy with steroids; neurological infection; therapy with antibiotics, pro-, pre- or metabiotics at the time of admission.
The age range was from 20 to 75 years (median: 56 (32–63) years); 17 study participants were female (58.6%) and 12 were male (41.4%). Among the pathologies included in the study were an acute cerebrovascular event (ACVE) (15 patients), severe traumatic brain injury (TBI) (7 patients), neurosurgery complications in the postoperative period (4 patients), anoxic brain injury after a successful CPR effort to reverse clinical death (3 patients). Ten patients were on MV and 19 had a tracheostomy tube. All patients were on enteral feeding; over half of them (n = 15) were fed through a nasogastric or gastrostomy tube (GT). Antibacterial therapy was administered to 21 patients who had urinary or lower respiratory tract infection (tab. 1). All patients received a metabiotic containing active metabolites of Bacillus subtilis, a sorbent and a prebiotic.
The level of consciousness was assessed using the Glasgow coma scale (GCS): 15 points — fully awake and aware, 14–13 points — clouding of consciousness, 12–9 points — sopor, 8–6 points — coma); antibiotic prescriptions were done on the day of blood/stool collection.
Sample collection
One stool sample and one venous blood sample were collected from each patient on the day of admission (prior to antimicrobial therapy) and then every 7 days until transfer from ICU. In total, 2 to 7 samples of biological specimens were obtained from each patient. Sample collection, transport and storage were performed following standard guidelines [20]. Stool samples were collected into a disposable sterile container. The containers were transported to the lab and stored at +2 – +8 °С until further use. Time from sample collection to sample analysis did not exceed 24 h. DNA was extracted from the supernatant: 0.1 g of the stool sample was mixed with 800 μl of the isotonic solution and vortexed until homogeneity. The resultant mixture was centrifuged at 12,045 g for 30 s. Further analysis was carried out following the protocol provided by the assay manufacturer (Colonoflor-16 by AlphaLab, Russia). Blood was collected from a venous catheter into an anticoagulant-free test tube. Serum samples were obtained by centrifuging the blood at 1,500 g for 10 min. Serum aliquots (500 μl) were poured into disposable Eppendorf tubes, frozen and stored at –20 °С until further use.
Analysis of gut microbiota taxonomic abundance
Composition of the gut microbiota was analyzed using Colonoflor-16 kits (AlphaLab; Russia), which include reagents for DNA extraction, PCR primers specific for all bacterial DNA (total bacterial mass) and species-specific primers. Measurements were performed using a CFX 96 Real-Time PCR Detection System (BioRad; USA).
Analysis of serum biomarkers
Concentrations of NSE, S100, procalcitonin (PCT), IL6, and cortisol were measured in 200 μl serum samples using reagent kits by Roche Diagnostics, Switzerland, and a Cobas e411 automated analyzer, which relies on the ElectroChemiLuminescence technology (Roche; Switzerland).
Albumin and CRP levels were determined using an automated AU 480 chemistry analyzer (Beckman Coulter; USA) and reagent kits by the same manufacturer.
Statistical analysis was carried out in Statistica 13.0 (Stat Soft Inc.; USA). The obtained data are presented below as median values and lower and upper quartiles. Intergroup comparisons were made using the Mann–Whitney U test. Correlation strength was measured using Spearman’s rank correlation coefficient. Differences were considered significant at p ≤ 0.05.
RESULTS
Our study included 29 patients (see tab. 1). Patients with etiologically different severe CNS injury formed a single group due to similar CCI manifestations regardless of the underlying condition. No significant differences in the taxonomic abundance of the gut microbiota were observed between the subgroups of patients receiving or not receiving antimicrobial therapy (:tab_2). Therefore, the subsequent intergroup comparisons were based on neurologic assessment scores.
Based on the level of consciousness, the patients were divided into 2 subgroups [21] (see tab. 1): vegetative state or minimally responsive patients (n = 10) and conscious patients with pronounced cognitive and mental impairment (n = 19). The latter is a clinical sign of CCI. The subgroups were comparable in terms of age (tab. 3).
The following features of the gut microbiota taxonomy were identified during the analysis aided by real-time PCR (fig. 1):
– total bacterial mass was comparable to the reference ranges provided by the manufacturer of the assay;
– gut microbiota of CCI patients contains Clostridium spp., Fusobacterium nucleatum, and Parvimonas micra, which are not detected in healthy individuals;
– for 6 microorganisms (Klebsiella spp., Candida spp., Staphylococcus aureus, Proteus spp., Enterobacter spp./ Citrobacter spp.), base 10 logarithms of CFU concentrations (lg CFU/G) were 1.5–2 times above the upper reference limit;
– Bacteroides thetaiotimicron were low in comparison with the reference values;
– significant differences were observed in F. prausnitzii concentrations between the subgroups of patients with different level of consciousness (p = 0.015);
Levels of serum biomarkers (cortisol, albumin, CRP, CRP to albumin ratio) differed significantly between the groups (see tab. 3). PCT was within the reference range in both conscious and vegetative state patients, but the differences between these two groups were significant (p = 0.009) (see tab. 3).
Based on their GCS scores, our patients were divided into 3 subgroups: 15 points — fully awake and aware patients (n = 37), 14–13 points — clouding of consciousness (n = 33), 12–6 points — sopor/coma (n = 40). Differences in the CRP/albumin ratio (fig. 2), cortisol and IL6 (fig. 3) observed between these groups were statistically significant.
To test correlations in the gut-brain axis system, we applied the correlation analysis to the levels of serum biomarkers and gut microbiota representatives. In vegetative state patients, significant negative correlations were discovered for cortisol and F. prausnitzii (r = –0.62) and cortisol and B. thetaiotaomicron (r = –0.57). In conscious patients, the CRP/albumin ratio value was directly correlated with the abundance of S. aureus (r = 0.72); S100 levels were directly correlated with B. fragilis group/F. prausnitzii (r = 0.45).
A strong negative correlation was discovered between the abundance of Enterococcus spp. and GCS scores (r = –0.77; р ≤ 0.05).
DISCUSSION
Based on our findings, we conclude that the taxonomic abundance of the gut microbiota in CCI patients differs significantly from the reference values, regardless of their neurological status. Pronounced dysbiosis in patients with CCI is characterized by increased levels of Proteobacteria, Candida spp., opportunistic Firmicutes and Bacteroides, and reduced abundance of commensal microorganisms (see fig. 1) producing low molecular weight metabolites (F. prausnitzii). These data are consistent with the findings of previous gut microbiota studies that employed 16S RNA sequencing and were conducted in patients undergoing intensive care [22] and critically ill patients with brain injury [23]. It is reported that pronounced dysbiosis developed by stroke survivors and manifested as the dominance of short-chain fatty acids producers, including Odoribacter and Akkermansia, was correlated with their clinical outcomes [24]. However, the cited study evaluated the composition of the gut microbiota in the first 48h after stroke, and we were unable to find any publications describing microbiota composition in chronically ill neurological patients; these facts underscores the novelty of our findings.
Biomarker analysis uncovered significant differences in the levels of cortisol, CRP, albumin and the CRP/albumin ratio between conscious and vegetative state patients (see tab. 3). The studied biomarkers were significantly correlated with the abundance of some gut microbiota representatives (see tab. 3), supporting the gut-brain axis concept. The established negative correlations between cortisol levels and the abundance of F. prausnitzii and В. thetaiotaomicron in vegetative state patients are consistent with earlier reports demonstrating that a decline in the abundance of the foregoing taxa compromises the neuroprotective function of the gut microbiota and therefore leads to nervous tissue injury [25], an immune response shift towards inflammation and autoimmunity [26]. In turn, elevated cortisol exerts a negative effect on the metabolism of critically ill patients, shifting the balance towards catabolism and promoting the hypermetabolism-hypercatabolism syndrome [27, 28].
In our study, CRP and cortisol levels, as well as the CRP/albumin ratio value, were twice as high in vegetative state patients, whereas their albumin levels were lower than in conscious participants, indicating more pronounced inflammation and catabolism in vegetative state. РСТ levels were within the reference range in both groups, although the difference between the groups was significant, suggesting aseptic inflammation unrelated to systemic bacterial infection (see tab. 3).
The CRP/albumin ratio is widely studied as a combined marker of systemic inflammation and nutritional status and also as an independent prognostic marker in patients with infection, malignancies or undergoing intensive care [10, 29, 30]. We have demonstrated that the CRP/albumin ratio indicates the severity of consciousness impairment on GCS in critically ill patients (see fig. 2) and correlates with the abundance of opportunistic S. aureus in the gut microbiota of such patients. S100 and NSE are traditionally used as markers of acute nervous tissue injury [31]. In our CCI patients, their only slightly changed levels were within the reference range, which might be reflective of the perpetuation of brain tissue injury. The observed direct correlation between S100 levels and the Bacteroides fragilis to Faecalibacterium prausnitzii ratio (a potential marker of proinflammatory type dysbiosis) and the strong negative correlation between GCS scores and Enterococcus spp. abundance confirm the association between dysbiosis and brain dysfunction. However, the link between the taxonomic abundance of the gut microbiota, the levels of neurologic biomarkers and the degree of neurologic deficit is not fully clear and needs to be further elucidated. We found no data on the correlation between the studied taxa and the levels of serum biomarkers in patients with CCI in the available literature.
To sum up, the gut microbiota of chronically critically ill patients with brain dysfunction undergoes certain transformations and can be deemed a “damaged organ”. The small study sample size and different therapeutic regimens did not allow us to assess the contribution of antibiotics to the changes in the gut microbiota. However, a complex approach based on the use of state-of-the-art technologies might help to optimize antibiotic therapies in the future.
CONCLUSIONS
1. Regardless of CNS injury severity, patients with CCI have pronounced imbalances in the taxonomic composition of their gut microbiota.
2. The established significant correlations between the studied serum biomarkers, GCS scores and certain gut microbiota representatives support the gut-brain axis concept.
3. Complex PCR-based diagnostics allows performing rapid dynamic assessment of the taxonomic composition of the gut microbiota.
4. Further research is needed to confirm the role of the gut microbiota in the pathogenesis of neurological deficit. Microbiota-targeted therapies might improve the efficacy of neurorehabilitation even in patients with severe brain damage.