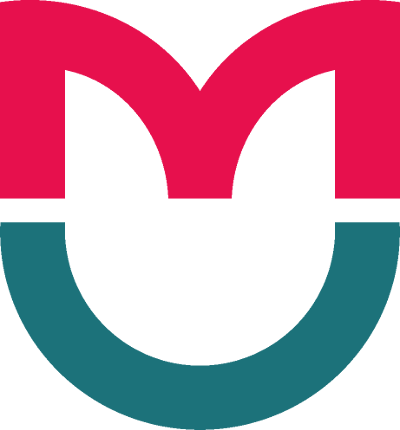
This article is an open access article distributed under the terms and conditions of the Creative Commons Attribution license (CC BY).
ORIGINAL RESEARCH
Significance of analysis of lipid extracts in cervical canal secretion for diagnosing of placenta-associated complications of pregnancy
Kulakov National Medical Research Center for Obstetrics, Gynecology and Perinatology, Moscow, Russia
Correspondence should be addressed: Natalia A. Lomova
Akademika Oparina, 4, Moscow, 117997, Russia; ur.xednay@avomol-ahsatan
Funding: the study was supported through the state assignment of the Ministry of Health of the Russian Federation [State registration number AAAA-A18-118053190026-6].
Acknowledgements: the authors are grateful to Dr. V. E. Frankevich, Head of the Department of Systems Biology in Reproduction of the V. I. Kulakov National Medical Research Center for Obstetrics, Gynecology and Perinatology, for his help in organization of the study, systematic analysis and manuscript editing.
Author contribution: Lomova NA — analysis of clinical data, systematic analysis, manuscript authoring; Chagovets VV — metabolic analysis (mass spectrometry), statistical analysis of the data obtained, manuscript editing; Tokareva AO — metabolic analysis (mass spectrometry), mass spectrometry data processing; Dolgopolova EL — collection and preparation of biological fluids, statistical analysis of the results; Karapetyan TE — analysis of clinical data, statistical analysis of the results; Magomedova AP — collection and preparation of biological fluids; Shmakov RG — analysis of clinical data, systematic analysis, manuscript editing.
Compliance with ethical standards: the study was approved by the ethical committee of Kulakov National Medical Research Center for Obstetrics, Gynecology and Perinatology (Minutes #11 of November 11, 2021), conducted in accordance with the requirements of the Declaration of Helsinki, International Conference on Harmonization (ICH), Standards of Good Clinical Practice (GCP), Federal Law "On the Basics of Health Protection of Citizens in the Russian Federation"; all patients signed a voluntary informed consent to participate in the study.
It has earlier been established that such common complications of pregnancy as Intrauterine Growth Restriction (IUGR) and preeclampsia (PE) are associated with placental dysfunction. With these pathologies, metabolic and circulatory systems fail to adapt to changes caused by pregnancy, which leads to hypertension and impaired placental blood flow. This not only causes undesirable consequences for the health of the mother and child during gestation, but can also affect their future health. In recent years, 10 to 20% of pregnancies in the economically developed countries have been complicated by some form of hypertension [1]. Preeclampsia is a pregnancy complication that occurs in the placenta and negatively affects both the mother and the fetus. PE is diagnosed in 5–7% of pregnant women worldwide [2]. This condition can develop after 20th week of pregnancy; its manifestations are hypertension, proteinuria and edema. Subsequently, it causes fetal distress and diminishes the chances of positive pregnancy outcome. IUGR prevents the fetus from realizing its growth potential in full: the weight and the body mass index (BMI) of the newborn are abnormal for the respective number of gestational weeks. About 4–8% of newborns are diagnosed with IUGR in industrialized countries, and in the developing countries this figure ranges from 6 to 30% [3, 4]. The causes of IUGR are distinguished into internal, peculiar to the fetus or placenta, and external, the mother-side factors affecting mainly the placenta and the fetus. There are also combinations of internal and external IUGR causes.
To date, there have not been developed adequate methods of prediction of these pregnancy complications, which obviously necessitates development of non-invasive and objective methods of prenatal diagnostics and monitoring.
Today, post-genomic omics technologies, including metabolomics and lipidomics, are growing rapidly and become more and more common in the routine clinical practice. With regard to the pathologies considered in this work, significant changes in the levels of metabolites were found even before preeclampsia manifested clinically, and these changes increase with the progression of the disorder [5–7]. Metabolomics can help detect the specific features of these conditions at the molecular level and aid in identification of the biological mechanisms underlying them, as well as in discovery of the new biomarkers. Metabolomics also offers a unique set of tools enabling identification of various endotypes of pathological conditions [7, 8] and diagnosing of different complications of pregnancy. Similar observations have been made for IUGR [9]. In the aforementioned works, researchers analyzed blood metabolome. Blood sampling is an invasive method of obtaining biological material. This study discusses the possibility of using cervical canal secretion to make PE or IUGR development prediction non-invasive.
Currently, the search for non-invasive and minimally invasive diagnostic markers in obstetrics is a promising area of scientific research. Cervical canal is one of the potential loci for minimally invasive sampling of biological material. There have been described potential biomarkers of spontaneous preterm labor that were identified through targeted proteomic analysis of cervicovaginal fluid samples from asymptomatic high-risk women. The proteins in cervicovaginal fluid of patients that experienced spontaneous preterm labor are extracellular matrix proteins that can also regulate the physiology of cell membranes [10]. In 2020, a statistically significant difference in the level of cytokines from cervicovaginal fluid samples, especially IL6 and IL17α, was found in the group of women with preterm labor and premature rupture of the membranes in the history. These indicators may be more accurate prognostic markers of preterm labor than fetal fibronectin that is the standard indicator relied on in clinical practice today [11]. During pregnancy, dysbiosis of the vaginal microbiota directly affects metabolic profiles, which can trigger premature birth. In 2020, researchers used nuclear magnetic resonance spectroscopy to analyze metabolic profile of cervicovaginal fluid and identified metabolic markers that can help predict preterm labor. The assessment of the ROC curve showed that acetone, ethylene glycol, formate, glycolate, isopropanol, methanol, and trimethylamine oxide were the best in the matter of predicting preterm labor. These metabolites can be useful markers clinically and for prognosis of preterm labor [12]. Thus, to date, it has been proven that the cervicovaginal fluid, which is part of the mother-placenta-fetus system, contains a number of biologically active components that can potentially be used for predicting and diagnosing obstetric pathology.
There is a wide range of methods applied to identify and validate biomarkers in metabolomic studies: ultraviolet and infrared spectroscopy, nuclear magnetic resonance spectroscopy, electrophoresis. However, the most common are metabolomic platforms based on liquid chromatography with mass spectrometric detection (LC-MS).
Metabolomics as part of studies of obstetric syndromes associated with placental insufficiency (PE and IUGR) can greatly facilitate understanding of the pathogenesis of these complications of pregnancy and development of new diagnostic and prognostic approaches.
The purpose of the study was to search for differences in the lipids of cervical canal secretion of patients with normal pregnancy and patients with fetal growth retardation and preeclampsia, as well as to develop mathematical models based on the differences found with the aim to create noninvasive methods for antenatal diagnosis of PE and IUGR .
METHODS
This was a case-control study conducted at the Kulakov National Medical Research Center for Obstetrics, Gynecology and Perinatology (Russia) from January to December 2020. The study involved 57 pregnant women, observed and helped to deliver within the study timeframe in the Center. The women were divided into three groups: Group I — 18 patients with preeclampsia, Group II — 19 patients with IUGR , Group III — 20 pregnant women without these complications, conditionally healthy (control group). Group I inclusion criteria: SBP ≥ 140 mmHg and/or DBP ≥ 90 mmHg after 20th week of pregnancy regardless of the history of blood pressure, in combination with proteinuria ≥ 0.3 g per day or ≥ 0.3 g/l in two portions of urine taken 6 hours apart. Group II inclusion criteria: IUGR diagnosed through a ultrasound study, if there was a slowdown in the rate of increase of the estimated fetal weight (EFW) and/or abdominal circumference (AC) < 10th percentile, in combination with pathological blood flow as shown with Doppler ultrasonography or EFW and/or AC values < 3rd percentile. Exclusion criteria: multiple pregnancy, cervical pathology, diabetes mellitus, impaired renal function, chronic arterial hypertension, oncological and infectious-inflammatory diseases during this pregnancy. This study did not include the cases of combined PE and IUGR. All patients delivered by caesarean section.
Collection and preparation of samples
On the day of admission to the Center, all pregnant women included in the study had their cervicovaginal secretion sampled before vaginal examination. To detect any infectious and inflammatory diseases of the urogenital tract, a smear was taken to established cleanliness and microbiological composition of the vagina. On average, the samples were taken 10–14 days before delivery.
The cervicovaginal secretion samples were collected with a disposable vaginal elevator and sterile disposable cytobrushes. After introduction of the elevator and visual identification of the external canal of the cervix, a cytobrush was inserted into the cervical canal to a depth of 0.5 cm and, after a clockwise rotation, removed in such a way as to avoid contact with the walls of the vagina and external genital organs. After collection the samples were cooled in liquid nitrogen and stored at –80 °C.
Before analysis, the samples were subjected to liquid-liquid extraction to obtain the lipid fraction. For this purpose, 500 μL of an H2O/methanol (1:1) solution were added to the test tube with the cytobrush, then mixed thoroughly for 5 minutes and sonicated for another 5 minutes. After that, the cytobrush was removed from the test tube, 1 ml of chloroform added thereto and the tube stirred for 10 minutes. Next, it was centrifuged for 5 minutes at 13000 G at ambient temperature, 925 μL of the lower layer taken, dried in a nitrogen flow and redissolved in 200 μL of isopropanol/acetonitrile (1:1) solution for further analysis.
Mass spectrometric analysis of lipid extracts
Lipid extracts were analyzed in a Dionex UltiMate 3000 liquid chromatography system (Thermo Scientific; Germany) connected to a MaXis Impact qTOF mass analyzer with an electrospray ionization source (Bruker Daltonics; Germany). Samples were separated by reversed-phase chromatography in a Zorbax C18 column (150 × 2.1 mm, 5 μm; Agilent, United States) with a linear gradient from 30 to 90% of eluent B in 20 minutes. Eluent A was a 60:40 acetonitrile/water solution with some 0.1% formic acid and 10 mmol/L ammonium formate; eluent B was a 90:8:2 isopropanol/acetonitrile/water solution with some 0.1% formic acid and 10 mmol/L ammonium formate. The elution flow rate was 40 μL/min, and the volume of the injected sample was 3 μL. The mass spectra were obtained in the positive and negative ions mode in the range of m/z 100–1700 Da, with the following settings: voltage across the capillary at 4.1 kV in the positive ion mode and –3.2 kV in the negative ion mode; pressure of the sputtering gas at 0.7 bar, drying gas flow rate 6 L/min, drying gas temperature 200 °C.
The initial mass spectrometric data were processed with the help of msConvert software from the Proteowizard 3.0.9987 package [13], MzMine [14]. Lipid identification was performed with LipidMatch scripts [15] using the exact mass and characteristic tandem mass spectra (MS/MS). The list of lipids is consistent with LipidMaps [16]
Statistical analysis
We used scripts written in the R language version 3.3.3 [17] and RStudio 1.383 [18] to process the results statistically.
The analyzed parameters were checked against the law of normal distribution with the help of Shapiro–Wilk test. When the distribution of the examined value was normal, we used the Student's t test for statistical analysis, and for the abnormal distribution cases we relied on the Mann-Whitney test for pairwise comparisons. To describe the quantitative data with normal distribution, we used arithmetic mean (M) and standard deviation (SD) in the M (SD) format. Features with distribution different from normal were described as a median (Me) and quartiles Q1 and Q3 in the Me format (Q1; Q3). The value of the threshold significance level p was taken at 0.05.
We developed the logistic regression models in order to assess the possibility of classifying patients into groups. Lipids showing statistically significant differences in levels between the groups were independent variables in the models. Akaike information criterion (AIC) enabled selection of the variables [19]. We continued selecting variables in stages while this action resulted in an increase in AIC. The quality of the resulting diagnostic model was tested by internal crossvalidation with control for individual objects. The values of the area under the operating curve, sensitivity and specificity were used for assessment. The patient's group membership was the dependent variable. For each model, we determined the Wald test, 95% confidence interval (CI), odds ratio (OR) and its confidence interval. The quality of the developed models was determined by constructing the ROC curve, determining the area under the ROC curve, and calculating the sensitivity and specificity.
RESULTS
Table 1 (tab. 1) gives the results of analysis of the clinical parameters of the groups.
It was found that the BMI of pregnant women from the IUGR group is significantly lower than that of women in the "normal" and "preeclampsia" groups (p = 0.005 and p = 0.0045, respectively).
All women delivered in the third trimester of pregnancy. The delivery time for the PE and IUGR groups significantly differed from that for the control group (p < 0.001), which is explained by the need for accelerated delivery based on obstetric indications. The share of preterm delivery in the PE group was 33.3% (six cases), in the IUGR group — 31.6% (six cases).
The average weight of newborns in the control group was 3493.6 g, compared to 2341 g and 2128.7 g in PE and IUGR groups, respectively (p ≤ 0.001).
It should be noted that, compared to the control group, the Apgar score in the PE group was significantly lower at the first minute of life (p = 0.04) and in the IUGR group — at the fifth minute (p = 0.05). One of the possible reasons behind the observations is that a newborn that suffered chronic hypoxia and growth retardation for a long period has the reserves of his/ her compensatory mechanisms depleted and experiences early neonatal adaptation in severe form. One child was diagnosed with moderate asphyxia at birth, which required additional respiratory support.
Analysis of lipid composition of the cervical canal secretion was enabled by LC-MS. LC-MS experiments were performed in both positive and negative ion modes, since this approach allows expanding the coverage of registered and identified lipids. LC-MS in positive ion mode allowed identifying 129 lipids, LC-MS in negative ion mode — 110 lipid compounds. Comparison of the relative lipid levels between the control group and the IUGR group revealed 10 lipids with levels differing significantly as registered in positive ion mode and 7 lipids with significantly different levels as registered in negative ion mode (fig. 1). The results of the comparison of control and PE groups were 1 lipid with significant level difference found in positive ion mode analysis and 2 such lipids discovered negative ion mode (fig. 2). As for the PE to IUGR groups comparison, there were two lipids the levels of which differed significantly (fig. 3). Mainly, the lipids were oxylipins (oxytriglycerides and oxycardiolipins), sphingomyelins, triglycerides, and cardiolipins.
Relying on the data obtained, we built the logistic regression models seeking to identify patients who may develop IUGR or PE, such identification based on the levels of lipids in the cervical canal secretion (tab. 2). A ROC analysis enabled assessment of quality of the built models; tab. 3 and Figures fig. 4 and fig. 5 present the results of this analysis. The models enabling allocation to the control and the IUGR groups had the best parameters; they made use of data obtained with both positive ion mode and negative ion mode. The AUC was 0.70 and 0.85, respectively. The model that enabled allocation to the PE and control groups had a rather high specificity (0.91) but low sensitivity (0.30). Thus, of the obstetric pathologies considered in this work (IUGR and PE), the non-invasive approach to lipid profiling based on the cervical canal secretion seems most promising for detection of IUGR. As for PE diagnosing, applicability of the described approach requires additional research. The IUGR /Normal and IUGR /PE models built on the positive ion mode and negative ion mode data can be considered for further improvement and validation.
DISCUSSION
The study allowed identifying 129 lipids with positive ion mode and 110 lipid compounds with negative ion mode; they are simple lipids, glycerides and complex lipids, which in turn are divided into glycerophospholipids and sphingolipids. The natural organic compounds of this large group, including fats and fat-like substances, participate in construction of cell membranes and regulation of metabolism. The group is undoubtedly interesting as a research subject in the context of obstetric pathologies. In particular, there may be value in investigation of changes in the group associated with the said pathologies and, specifically, tracking the fluctuations at the interface of the mother-placenta-fetus system. Fatty acids and lipids play a primary role in growth and development during the embryonic period. Unbalanced intake of fatty acids during the perinatal period alters the composition of fatty acids in the membrane phospholipids of the fetus, which can cause structural and functional problems in its cells. In addition, the metabolic and neuroendocrine environment of the fetus and the newborn plays a key role in the energy balance regulation. Lack of proper balance between fatty acids and lipids during pregnancy can lead to irreversible changes in the control of neuroendocrine function and energy metabolism in the fetus, leading to metabolic programming. Thus, control of the lipid levels enables detection of abnormalities in the motherplacenta-fetus system even at the preclinical stage [20]. It has been shown (in both animal and human experiments) that the metabolic pathway of sphingolipids plays a critical role in fetal and maternal tolerance, regulating innate immunity at the mother-fetus interface. These findings may help develop new therapeutic strategies for obstetric complications [21].
During pregnancy, metabolic changes occur in all systems and processes of the woman's body, including lipid metabolism. Cholesterol and free fatty acids are essential for cellular synthesis of embryonic membranes. The fetus synthesizes lipids and makes extensive use of maternal lipids, although free transplacental transport of maternal lipoproteins is limited by the "placental barrier" [22]. Phospholipids and triacylglycerols (TAGs) from the mother's body do not penetrate directly to the fetus. There is a number of mechanisms enabling transportation of fatty acids to the fetus; these mechanisms imply binding fatty acids [23] and transporting them intensively across the placenta. Circulating lipids have a variety of effects on the endothelial cell function. Dyslipidemia is often associated with dysfunction of these cells [24]. Several studies have shown a concentration-dependent relationship between elevated TAG levels and the risk of PE. There are many mechanisms that may be used as explanation of the correlation between dyslipidemia and PE. For example, increased levels of lipid fractions (such as TAG) trigger their accumulation in endothelial cells and subdue production of prostacyclin, which subsequently leads to endothelial dysfunction [25]. In our study, the level of TG 18: 1_24: 0_24: 1, CL 16: 0_16: 1_22: 6_22: 6, PC O-22: 1/18: 1 had significant differences in the PE-Normal groups comparison (p = 0.008, p = 0.02, p = 0.04, respectively). The specificity achieved for the PE/Normal diagnostic model is 0.91 (cut-off threshold — 0.10; AUC — 0.67).
In case of IUGR, placenta does not function properly and has its structure compromised, and the manifestations of the problem are detectable with the fetus but not with the mother's body. Several researchers [26, 27] have found that during pregnancy complicated by IUGR, there is a decrease in the level of lipids in the umbilical cord blood and the mother's blood, and the number of their receptors goes down, too. Low levels of lipids and their receptors in the feto-placental complex will probably have very serious consequences for the normal growth and development of the fetus, since they will only insufficiently enable transport of cholesterol, normal functioning of the placenta and harmonious growth of the fetus. As we have registered in our study, the levels of 17 lipids (tab. 2) were significantly different in the IUGR and the Normal groups (p < 0.05). The specificity achieved for the PE/Normal diagnostic model is 0.91, sensitivity is 0.81 (cut-off threshold –0.50; AUC — 0.85).
The significant difference in the results of examination of cervicovaginal secretion samples taken in the IUGR and PE groups (p = 0.04) is of particular interest. This difference clearly demonstrates that these pregnancy complications, initially considered to stem from the common placental dysfunction, are not the same on the pathogenetic level.
CONCLUSIONS
Our study clearly demonstrates the possibility of using cervicovaginal fluid in complex diagnostic monitoring of pregnant women with placenta-associated obstetric complications. We performed an in-depth clinical-anamnestic and massspectrometric analysis of samples taken from 57 pregnant women. Analysis of the cervical canal secretion of pregnant women with IUGR and PE revealed 129 lipid compounds when using LC-MS in positive ion mode and 110 lipid compounds with LC-MS in negative ion mode. The level of a number of lipids differed significantly between the PE and Normal groups and the IUGR and Normal groups. This observation may indicate the existing differences in the chain of biological processes of the pathogenesis of these pregnancy complications that are commonly believed to stem from placental dysfunction. Further research in this direction may help identify new approaches to the development of targeted therapy for the "major obstetric syndromes." The lipids were mainly oxylipins (oxytriglycerides and oxycardiolipins), sphingomyelins, triglycerides, and cardiolipins, which means this method may be used as part of a complex of predictive measures. Based on the differences identified, we built logistic regression models that can contribute to the development of methods of non-invasive diagnostics of these diseases. The result of our effort is the clear demonstration of the possibility to determine the level of lipids in the cervicovaginal fluid noninvasively in the context of complex diagnostic monitoring of pregnant women with placenta-associated obstetric complications.