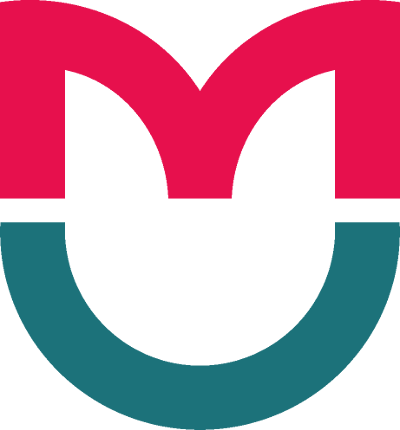
METHOD
Sensors for analysis of drugs, drug-drug interactions, and catalytic activity of enzymes
1 Institute of Biomedical Chemistry (IBMC), Moscow, Russia
2 Pirogov Russian National Research Medical University, Moscow, Russia
Correspondence should be addressed: Lyubov E. Agafonova
Pogodinskaya, 10/8, Moscow, 119121, Russia; ur.liam@abulavonofaga
Funding: the study was carried out within the framework of the Russian Federation fundamental research program for the long-term period for 2021–2030.
Author contribution: Agafonova LE — experimental procedure, data processing, manuscript writing, building graphs; Bulko TV — sample preparation, experimental procedure; Kuzikov AV — statistical data processing, manuscript writing; Masamrekh RA — sample preparation, experimental procedure; Shumyantseva VV — concept, manuscript writing, data analysis.
Electrochemical method of analysis is a powerful tool to estimate the drug content and purity, as well as the drug concentration both in pharmaceutical fluids and in biological fluids or tissues (urine, blood serum, blood plasma, whole blood, cell lysates). Despite the use of various drug evaluation methods (such as spectrophotometry, colorimetry, fluorescence spectroscopy, gas chromatography–mass spectrometry, high-performance liquid chromatography (HPLC), thin-layer chromatography, titrimetry, capillary electrophoresis, high-performance liquid chromatography–tandem mass spectrometry and thermogravimetric analysis, radiometry, immunoassay) [1], electrochemical techniques are in demand as well due to high sensitivity, unique electrochemical signatures of the relevant compounds, reasonable cost, fast electrochemical analysis speed, low sample volumes (2–60 µL), and portable equipment. Electroanalysis allows for simultaneous detection of several pharmaceuticals and makes it possible to assess these drugs by various electrochemical methods in order to improve the test sensitivity (cyclic and stripping voltammetry, square-wave voltammetry, differential pulse voltammetry, chronoamperometry, and electrochemical impedance spectroscopy) [2–6].
Cytochrome Р450 is a superfamily of heme-thiolate monooxygenases involved in metabolism of xenobiotics and endogenous compounds [7]. The method for measuring catalytic activity of this hemoprotein class by electrochemical oxidation of substrate drugs performed well in the previously published study [4], aimed at measuring the catalytic activity of cytochrome Р450 3А4. Cytochrome Р450 19A1 (CYP19A1, aromatase) is a key enzyme for estrogen biosynthesis [8]. Electrochemical methods for determination of estrone or β-estradiol using the electrodes, modified with various nanocomposites, had been previously developed in order to quantify the products of the CYP19A1-dependent electrocatalytic reaction [9–12]. Electrochemical oxidation of estrone or β-estradiol, being the aromatase metabolites, could be also detected on the commercially available three-prong printed graphite electrodes (PGE) [13]. Electrooxidation of (S)-7-hydroxywarfarin, being the cytochrome Р450 2С9 metabolite, was used to evaluate the kinetic parameters of this hemoprotein [5].
The study was aimed to develop the reagentless electrochemical method for drug identification and quantification under physiological conditions in order to provide the possibility of assessing drugs in blood serum using the commercially available screen-printed three-prong electrodes with the graphite working electrode.
METHODS
Electrochemical measurements were performed with the PGSTAT 12 Autolab and PGSTAT 312N Autolab potentiostats (Metrohm Autolab Ins.; Netherlands) and the GPES and NOVA software, versions 4.9.7 and 2.0, respectively (Netherlands). The three-prong printed graphite electrodes (PGE, ColorElectronics; Russia) were used, together with the graphite working and auxiliary electrodes, and the silver chloride reference electrode. The diameter of working electrode was 0.2 cm (the area was 0.0314 cm2). All potentials were referred to the silver/silver chloride reference electrode (Ag/AgCl).
The following reagents were used in the study: monobasic potassium phosphate (Reachem; Russia), sodium chloride (Reachem; Russia), diclofenac sodium (substance, Sigma-Aldrich; India), ibuprofen sodium (substance, SigmaAldrich; India), acetaminophen (pharmaceutical dosage form, Pharmstandard; Russia), mexidol (pharmaceutical dosage form, Pharmasoft; Russia), serum (S 1005-14, UsBiological; USA).
The 10 mM stock solutions were prepared in the 0.1 М potassium phosphate buffer (рН 7.4), containing 0.05 М NaCl, the desired concentrations were obtained by diluting with buffer and stored at +4 °С.
The real-time measurements were performed by differential pulse voltammetry (DPV) over the potential range of (0–1.6) V with the 0.01 V potential step and 25 Hz frequency, and by cyclic voltammetry (CV). Experiments were performed under aerobic conditions at room temperature. A total of 60 µL (volume essential for uniform distribution of the drop across the electrodes) of the drug solution to be analyzed were applied to the surface of the disposable PGE, covering the working electrode, auxiliary electrode, and reference electrode [14]. At least three electrodes were used to assess the results repeatability for each concentration.
Peak current of analyte oxidation was plotted against analyte concentration in order to calculate sensitivity and the detection limit. The resulting calibration dependencies were used to caclulate sensitivity (equation 1) and the detection limit (equation 2) [15]:
where S — sensitivity, I — current, C — drug concentration, Сlim — detection limit, σ — residual standard deviation (standard deviation of the regression coefficient b).
To remove protein components from blood serum, 2.5 mL of blood serum were collected in the 10 mL glass tube and added 2.5 mL of the 15% (w/v) acetonitrile solution of zinc sulfate (50/40, v/v) or 10% trichloroacetic acid (1 : 10). The test tube was shaken for 20 min and equilibrated at 4 °C for 15 min, then the solution was centrifuged at 13,500 rpm for 5 min. Subsequently, supernatant was discarded, and the solution was used for further analysis [16]. After protein precipitation, blood serum was diluted 10 times with the 0.1 М potassium phosphate buffer, containing 50 mМ NaCl, рН 7.4.
RESULTS
Rational selection of the electrode type is the key point of electrochemical analysis, which is essential for the most effective electron transfer and detection of molecules, biochemical events, and catalytic current, being the indicator of electrocatalysis [17]. It is shown, that modification of the electrode working surface with nanomaterials (carbon nanotubes, graphene, graphene oxide, metal nanoparticles) contributes to the increased sensor analytical sensitivity [6]. However, modified PGE may acquire background characteristics that impede direct registration of electrooxidation/electroreduction [18]. In unmodified PGE, background characteristics in the electrolyte buffer show no unintended additional signals, and the current values registered are rather low [5]. Furthermore, unmodified electrodes are commercially available, reproducibility of electrochemical analysis is high, which constitutes an essential element of the further sensors' practical utilization for drug analysis and purity evaluation, particularly in the clinical diagnostics laboratories. In this regard, we have developed methods for drug detection using precisely the unmodified graphite working electrode surfaces.
Electroanalytical characteristics of pharmaceuticals
N-acetyl-para-aminophenol, or drug А is an antipyretic and analgesic agent commonly used in patients with mild to moderate pain or to lower the body temperature, including in viral and bacterial infections [3]. It has been shown that N-acetyl-p-benzoquinone imine (NAPQI) is the main product of the drug A oxidation [3]. The mechanism, underlying the analgesic effect, is associated with prostaglandin synthesis inhibition in the central nervous system [2, 3, 19].
Fig. 1 (fig. 1) provides the differential pulse voltammogram of the 1 mМ drug А acquired on the unmodified PGE in the potential range of (0–1.2) V. Under aerobic conditions, drug A oxidation occurs at the Eox potential of (0.50 ± 0.03) V (vs. Ag/AgCl). The figure insert shows the linear growth of the drug A oxidation peak current with the concentration increase from 0.05 mМ to 1.00 mМ. The drug A oxidation potential is stable within the margin of error.
Table (table) provides electroanalytical characteristics (concentration range, oxidation potential), equation for the relationship between the oxidation peak current and the concentration, R2 coefficient of determination, as well as the electrochemical system sensitivity, calculated using equation 1, and the detection limit of drug A, calculated using equation 2.
(RS)-2-(4-isobutylphenyl)-propionic acid, or drug I is a non-steroidal anti-inflammatory drug from the group of the propionic acid derivatives, which possesses analgesic and antipyretic activity [12, 13]. Fig. 2 (fig. 2) provides the drug I differential pulse voltammograms acquired on PGE in the potential range of (0.6–1.6) V and the concentration range of (0.5–10) mМ. Electrochemical oxidation of the 1 mМ drug I occurs at high potential values (1.29 ± 0.02) V (see table).
(2-(2,6-dichloroanilino)-phenylacetic acid, or drug D is a non-steroidal anti-inflammatory drug from the group of the phenylacetic acid derivatives. The sodium salt is used in the pharmaceutical dosage forms. The drug has multiple trading names, it is prescribed to patients with many disorders, such as rheumatoid arthritis, osteoarthritis, various inflammation conditions [20, 21]. The drug has analgesic, anti-inflammatory, and anticancer effects [21]. Drug D undergoes extensive metabolism, mediated by glucurosyltransferase, to form diclofenac acyl glucuronide. On exposure to cytochrome Р450, drug D undergoes oxidative metabolism to form 4'-hydroxydiclofenac (catalyzed by cytochrome Р450 2С9) and 5-hydroxydiclofenac (catalyzed by cytochrome Р450 3А4) [22, 23].
In some cases, the drug can cause unwanted side effects: stomach hemorrhage, high blood pressure in patients with Shy–Drager syndrome and diabetes mellitus. The long-term use may result in infarction or stroke [20]. That is why the drug D analysis remains an ongoing challenge posed by bioanalytical and pharmaceutical chemistry.
Fig. 3 (fig. 3) provides the drug D differential pulse voltammograms acquired on PGE in the potential range of (0.2 – 1.0) V and the concentration range of (50 – 500) µM. The oxidation potential of (0.57 ± 0.05) V was stable within the margin of error in the studied concentration range. The linear relationship was found between the oxidation peak current and the drug D concentration (see table).
DISCUSSION
CV in the scan rate range of (0.05–0.18) V/s was used to characterize the drug electrochemical oxidation processes. The CV results showed the linear relationship between the oxidation peak currents of the 1 mМ drug А, 100 µМ drug D and 5 mМ drug I, and the scan rate square root ν1/2 (Fig. 2 (а), 4 (а), 6 (а), Appendix), reflecting the diffusion-controlled electrochemical oxidation of the drugs on the unmodified PGE in the studied range of the potential scan rates [24]. There was also a linear relationship between the oxidation peak potentials and the logarithm of scan rate, log ν (Fig. 2 (b), 4(b), 6(b), Appendix), typical for irreversible electrochemical processes. This is confirmed by the CV technique: only the oxidation peaks of the 1 mМ drug А, 100 µМ drug D and 5 mМ drug I are observed on the unmodified PGE (Fig. 1, 3 and 5, Appendix), suggesting the irreversible nature of electrochemical reactions. These results are in line with the mechanisms of the studied drugs electrochemical oxidation [2, 3, 6, 19–22, 25–30].
Polypharmacy, i.e. prescribing several medications to one patient, is widely used in clinical pharmacology and therapeutics. Pharmacokinetic and pharmacodynamic parameters should be monitored in multimorbid patients (prescribed two or more medications). Electroanalysis based on the drug electrochemical oxidation registration allows for simultaneous detection of several compounds. This approach can be used to determine the drugs' mutual influence. Thus, antioxidant metabolic drug mexidol (2-ethyl-6-methyl-3-hydroxypyridine succinate, drug М), which is prescribed to improve cerebral circulation, is commonly used in various groups of patients with comorbidities. PGE were used to analyze the drug combinations D + М (fig. 4), D + I (fig. 5). Electrochemical oxidation of drug М is detected at the potential of +0.67 ± 0.06 V (fig. 4). However, drug М (98 µМ) has virtually no effect on the drug D (100 µМ) electrochemical oxidation. There is a slight shift of the drug D oxidation potential by (5 ± 2) mV toward the more positive (anodic) area, which is indicative of the more complicated electrochemical oxidation process. The oxidation peak current is slightly reduced (± 6%), which allows for the drug D quantification in the presence of drug М (fig. 4.). Fig. 5 (fig. 5) provides DPV for the combination of the 25 µМ drug D and 200 µМ drug I acquired on the PGE. The measurements have been performed over the potential range of (0–1.6) V. Electrooxidation peaks of the drugs D (Еоx = +0.54 ± 0.02 V) and I (Еоx = +1.29 ± 0.02 V) are clearly distinguished, however, the concentration dependence remains.
Drug D was also determined by electrochemical oxidation in the blood serum samples. The concentration of drug D added to blood serum was 100 µМ, and the oxidation peak current recorded was 7.5 × 10–8 А (fig. 3). According to the insert in the fig. 3 and the calibration dependence (see table), the drug D concentration calculated using the regression equation was (95 ± 5) µМ.
CONCLUSIONS
The method for drug analysis using the commercially available disposable unmodified screen-printed electrodes has been developed. Sensitivity of the method corresponds to the clinically relevant concentration ranges. Electrooxidation of pharmaceuticals using electrodes as measuring tools is an effective analytical approach to the drug purity assessment and drug concentration determination, particularly in biological fluids used for therapeutic drug monitoring. The methods for drug quantification based on electrochemical oxidation may be used to assess catalytic activity of enzymes involved in the phase I metabolism, such as cytochrome Р450, flavin-containing monooxygenases, aldehyde oxidase, aldehyde dehydrogenase, alcohol dehydrogenase, carboxylesterase, and to assess activity of enzymes involved in the phase II metabolism, such as UDP-glucuronosyltransferases, sulfotransferase, glutathione transferase. The method can be also used for registration of the comparative kinetics of the polymorphic variants and genetically engineered mutant enzymes in order to develop the biocatalyst isoforms important in technology.